Inhibitory stabilization of the cortical network underlies visual surround suppression
- PMID: 19477158
- PMCID: PMC2691725
- DOI: 10.1016/j.neuron.2009.03.028
Inhibitory stabilization of the cortical network underlies visual surround suppression
Abstract
In what regime does the cortical circuit operate? Our intracellular studies of surround suppression in cat primary visual cortex (V1) provide strong evidence on this question. Although suppression has been thought to arise from an increase in lateral inhibition, we find that the inhibition that cells receive is reduced, not increased, by a surround stimulus. Instead, suppression is mediated by a withdrawal of excitation. Thalamic recordings and previous work show that these effects cannot be explained by a withdrawal of thalamic input. We find in theoretical work that this behavior can only arise if V1 operates as an inhibition-stabilized network (ISN), in which excitatory recurrence alone is strong enough to destabilize visual responses but feedback inhibition maintains stability. We confirm two strong tests of this scenario experimentally and show through simulation that observed cell-to-cell variability in surround effects, from facilitation to suppression, can arise naturally from variability in the ISN.
Figures
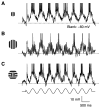
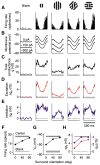
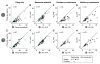
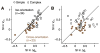
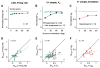
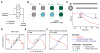
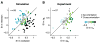

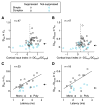
Similar articles
-
Orientation tuning of surround suppression in lateral geniculate nucleus and primary visual cortex of cat.Neuroscience. 2007 Nov 23;149(4):962-75. doi: 10.1016/j.neuroscience.2007.08.001. Epub 2007 Aug 9. Neuroscience. 2007. PMID: 17945429
-
Surround suppression sharpens orientation tuning in the cat primary visual cortex.Eur J Neurosci. 2009 Mar;29(5):1035-46. doi: 10.1111/j.1460-9568.2009.06645.x. Eur J Neurosci. 2009. PMID: 19291228
-
Temporal properties of spatial frequency tuning of surround suppression in the primary visual cortex and the lateral geniculate nucleus of the cat.Eur J Neurosci. 2010 Jun;31(11):2086-100. doi: 10.1111/j.1460-9568.2010.07235.x. Eur J Neurosci. 2010. PMID: 20604803
-
Contribution of feedforward, lateral and feedback connections to the classical receptive field center and extra-classical receptive field surround of primate V1 neurons.Prog Brain Res. 2006;154:93-120. doi: 10.1016/S0079-6123(06)54005-1. Prog Brain Res. 2006. PMID: 17010705 Review.
-
The dynamics of visual responses in the primary visual cortex.Prog Brain Res. 2007;165:21-32. doi: 10.1016/S0079-6123(06)65003-6. Prog Brain Res. 2007. PMID: 17925238 Review.
Cited by
-
Strength of gamma rhythm depends on normalization.PLoS Biol. 2013;11(2):e1001477. doi: 10.1371/journal.pbio.1001477. Epub 2013 Feb 5. PLoS Biol. 2013. PMID: 23393427 Free PMC article.
-
State modulation in spatial networks with three interneuron subtypes.bioRxiv [Preprint]. 2024 Aug 24:2024.08.23.609417. doi: 10.1101/2024.08.23.609417. bioRxiv. 2024. PMID: 39229194 Free PMC article. Preprint.
-
Surround suppression and temporal processing of visual signals.J Neurophysiol. 2015 Apr 1;113(7):2605-17. doi: 10.1152/jn.00480.2014. Epub 2015 Feb 4. J Neurophysiol. 2015. PMID: 25652919 Free PMC article.
-
Dynamic coding of signed quantities in cortical feedback circuits.Front Psychol. 2012 Aug 3;3:254. doi: 10.3389/fpsyg.2012.00254. eCollection 2012. Front Psychol. 2012. PMID: 22876235 Free PMC article.
-
Beta oscillations and waves in motor cortex can be accounted for by the interplay of spatially structured connectivity and fluctuating inputs.Elife. 2023 Mar 14;12:e81446. doi: 10.7554/eLife.81446. Elife. 2023. PMID: 36917621 Free PMC article.
References
-
- Akasaki T, Sato H, Yoshimura Y, Ozeki H, Shimegi S. Suppressive effects of receptive field surround on neuronal activity in the cat primary visual cortex. Neurosci Res. 2002;43:207–220. - PubMed
-
- Amit DJ, Brunel N. Model of global spontaneous activity and local structured activity during delay periods in the cerebral cortex. Cereb Cortex. 1997;7:237–252. - PubMed
-
- Anderson JS, Carandini M, Ferster D. Orientation tuning of input conductance, excitation, and inhibition in cat primary visual cortex. J Neurophysiol. 2000;84:909–926. - PubMed
Publication types
MeSH terms
Grants and funding
LinkOut - more resources
Full Text Sources
Other Literature Sources
Miscellaneous