Hypoxic preconditioning enhances the benefit of cardiac progenitor cell therapy for treatment of myocardial infarction by inducing CXCR4 expression
- PMID: 19407239
- PMCID: PMC2756190
- DOI: 10.1161/CIRCRESAHA.109.197723
Hypoxic preconditioning enhances the benefit of cardiac progenitor cell therapy for treatment of myocardial infarction by inducing CXCR4 expression
Abstract
Myocardial infarction rapidly depletes the endogenous cardiac progenitor cell pool, and the inefficient recruitment of exogenously administered progenitor cells limits the effectiveness of cardiac cell therapy. Recent reports indicate that interactions between the CXC chemokine stromal cell-derived factor 1 and its receptor CXC chemokine receptor 4 (CXCR4) critically mediate the ischemia-induced recruitment of bone marrow-derived circulating stem/progenitor cells, but the expression of CXCR4 in cardiac progenitor cells is very low. Here, we studied the influence of hypoxia on CXCR4 expression in cardiac progenitor cells, on the recruitment of intravenously administered cells to ischemic heart tissue, and on the preservation of heart function in a murine myocardial infarction model. We found that hypoxic preconditioning increased CXCR4 expression in CLK (cardiosphere-derived, Lin(-)c-kit(+) progenitor) cells and markedly augmented CLK cell migration (in vitro) and recruitment (in vivo) to the ischemic myocardium. Four weeks after surgically induced myocardial infarction, infarct size and heart function were significantly better in mice administered hypoxia-preconditioned CLK cells than in mice treated with cells cultured under normoxic conditions. Furthermore, these effects were largely abolished by the addition of a CXCR4 inhibitor, indicating that the benefits of hypoxic preconditioning are mediated by the stromal cell-derived factor 1/CXCR4 axis, and that therapies targeting this axis may enhance cardiac-progenitor cell-based regenerative therapy.
Figures
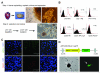
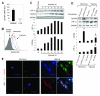
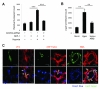
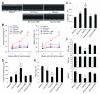
Comment in
-
Importance of the SDF-1:CXCR4 axis in myocardial repair.Circ Res. 2009 May 22;104(10):1133-5. doi: 10.1161/CIRCRESAHA.109.198929. Circ Res. 2009. PMID: 19461103 Free PMC article. No abstract available.
Similar articles
-
Hypoxic preconditioning improves survival of cardiac progenitor cells: role of stromal cell derived factor-1α-CXCR4 axis.PLoS One. 2012;7(7):e37948. doi: 10.1371/journal.pone.0037948. Epub 2012 Jul 18. PLoS One. 2012. PMID: 22815687 Free PMC article.
-
Myocardial CXCR4 expression is required for mesenchymal stem cell mediated repair following acute myocardial infarction.Circulation. 2012 Jul 17;126(3):314-24. doi: 10.1161/CIRCULATIONAHA.111.082453. Epub 2012 Jun 9. Circulation. 2012. PMID: 22685115
-
Bone-derived Nestin-positive mesenchymal stem cells improve cardiac function via recruiting cardiac endothelial cells after myocardial infarction.Stem Cell Res Ther. 2019 Apr 27;10(1):127. doi: 10.1186/s13287-019-1217-x. Stem Cell Res Ther. 2019. PMID: 31029167 Free PMC article.
-
SDF-1α as a therapeutic stem cell homing factor in myocardial infarction.Pharmacol Ther. 2011 Jan;129(1):97-108. doi: 10.1016/j.pharmthera.2010.09.011. Epub 2010 Oct 20. Pharmacol Ther. 2011. PMID: 20965212 Review.
-
Genetically manipulated progenitor/stem cells restore function to the infarcted heart via the SDF-1α/CXCR4 signaling pathway.Prog Mol Biol Transl Sci. 2012;111:265-84. doi: 10.1016/B978-0-12-398459-3.00012-5. Prog Mol Biol Transl Sci. 2012. PMID: 22917235 Review.
Cited by
-
Therapeutic Efficacy of Interferon-Gamma and Hypoxia-Primed Mesenchymal Stromal Cells and Their Extracellular Vesicles: Underlying Mechanisms and Potentials in Clinical Translation.Biomedicines. 2024 Jun 20;12(6):1369. doi: 10.3390/biomedicines12061369. Biomedicines. 2024. PMID: 38927577 Free PMC article. Review.
-
MiR-211/STAT5A Signaling Modulates Migration of Mesenchymal Stem Cells to Improve its Therapeutic Efficacy.Stem Cells. 2016 Jul;34(7):1846-58. doi: 10.1002/stem.2391. Epub 2016 May 19. Stem Cells. 2016. PMID: 27145179 Free PMC article.
-
Epigenetic regulation of cardiac progenitor cells marker c-kit by stromal cell derived factor-1α.PLoS One. 2013 Jul 24;8(7):e69134. doi: 10.1371/journal.pone.0069134. Print 2013. PLoS One. 2013. Retraction in: PLoS One. 2021 Feb 12;16(2):e0247094. doi: 10.1371/journal.pone.0247094 PMID: 23894420 Free PMC article. Retracted.
-
RNAase III-Type Enzyme Dicer Regulates Mitochondrial Fatty Acid Oxidative Metabolism in Cardiac Mesenchymal Stem Cells.Int J Mol Sci. 2019 Nov 7;20(22):5554. doi: 10.3390/ijms20225554. Int J Mol Sci. 2019. PMID: 31703292 Free PMC article.
-
Chemokine Receptors Expression in MSCs: Comparative Analysis in Different Sources and Passages.Tissue Eng Regen Med. 2017 Sep 19;14(5):605-615. doi: 10.1007/s13770-017-0069-7. eCollection 2017 Oct. Tissue Eng Regen Med. 2017. PMID: 30603514 Free PMC article.
References
-
- Rosamond W, Flegal K, Friday G, Furie K, Go A, Greenlund K, Haase N, Ho M, Howard V, Kissela B, Kittner S, Lloyd-Jones D, McDermott M, Meigs J, Moy C, Nichol G, O’Donnell CJ, Roger V, Rumsfeld J, Sorlie P, Steinberger J, Thom T, Wasserthiel-Smoller S, Hong Y. Heart disease and stroke statistics--2007 update: a report from the American Heart Association Statistics Committee and Stroke Statistics Subcommittee. Circulation. 2007;115:e69–171. - PubMed
-
- Beltrami AP, Barlucchi L, Torella D, Baker M, Limana F, Chimenti S, Kasahara H, Rota M, Musso E, Urbanek K, Leri A, Kajstura J, Nadal-Ginard B, Anversa P. Adult cardiac stem cells are multipotent and support myocardial regeneration. Cell. 2003;114:763–776. - PubMed
-
- Martin CM, Meeson AP, Robertson SM, Hawke TJ, Richardson JA, Bates S, Goetsch SC, Gallardo TD, Garry DJ. Persistent expression of the ATP-binding cassette transporter, Abcg2, identifies cardiac SP cells in the developing and adult heart. Dev Biol. 2004;265:262–275. - PubMed
-
- Matsuura K, Nagai T, Nishigaki N, Oyama T, Nishi J, Wada H, Sano M, Toko H, Akazawa H, Sato T, Nakaya H, Kasanuki H, Komuro I. Adult cardiac Sca-1-positive cells differentiate into beating cardiomyocytes. J Biol Chem. 2004;279:11384–11391. - PubMed
Publication types
MeSH terms
Substances
Grants and funding
LinkOut - more resources
Full Text Sources
Other Literature Sources
Medical
Research Materials