NADPH oxidase is required for the sensory plasticity of the carotid body by chronic intermittent hypoxia
- PMID: 19369559
- PMCID: PMC2692682
- DOI: 10.1523/JNEUROSCI.4768-08.2009
NADPH oxidase is required for the sensory plasticity of the carotid body by chronic intermittent hypoxia
Abstract
Respiratory motoneuron response to hypoxia is reflex in nature and carotid body sensory receptor constitutes the afferent limb of this reflex. Recent studies showed that repetitive exposures to hypoxia evokes long term facilitation of sensory nerve discharge (sLTF) of the carotid body in rodents exposed to chronic intermittent hypoxia (CIH). Although studies with anti-oxidants suggested the involvement of reactive oxygen species (ROS)-mediated signaling in eliciting sLTF, the source of and the mechanisms associated with ROS generation have not yet been investigated. We tested the hypothesis that ROS generated by NADPH oxidase (NOX) mediate CIH-evoked sLTF. Experiments were performed on ex vivo carotid bodies from rats and mice exposed either to 10 d of CIH or normoxia. Acute repetitive hypoxia evoked a approximately 12-fold increase in NOX activity in CIH but not in control carotid bodies, and this effect was associated with upregulation of NOX2 mRNA and protein, which was primarily localized to glomus cells of the carotid body. sLTF was prevented by NOX inhibitors and was absent in mice deficient in NOX2. NOX activation by CIH required 5-HT release and activation of 5-HT(2) receptors coupled to PKC signaling. Studies with ROS scavengers revealed that H(2)O(2) generated from O(2).(-) contributes to sLTF. Priming with H(2)O(2) elicited sLTF of carotid bodies from normoxic control rats and mice, similar to that seen in CIH-treated animals. These observations reveal a novel role for NOX-induced ROS signaling in mediating sensory plasticity of the carotid body.
Figures
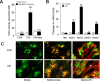
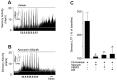
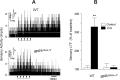
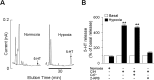
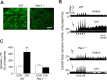
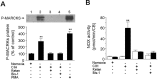
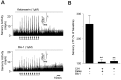
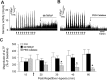
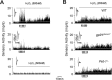
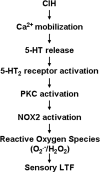
Similar articles
-
Heterozygous HIF-1alpha deficiency impairs carotid body-mediated systemic responses and reactive oxygen species generation in mice exposed to intermittent hypoxia.J Physiol. 2006 Dec 1;577(Pt 2):705-16. doi: 10.1113/jphysiol.2006.114033. Epub 2006 Sep 14. J Physiol. 2006. PMID: 16973705 Free PMC article.
-
Angiotensin II evokes sensory long-term facilitation of the carotid body via NADPH oxidase.J Appl Physiol (1985). 2011 Oct;111(4):964-70. doi: 10.1152/japplphysiol.00022.2011. Epub 2011 Jun 2. J Appl Physiol (1985). 2011. PMID: 21636565 Free PMC article.
-
Sensory plasticity of carotid body is correlated with oxidative stress in paraventricular nucleus during chronic intermittent hypoxia.J Cell Physiol. 2019 Aug;234(8):13534-13543. doi: 10.1002/jcp.28031. Epub 2019 Jan 4. J Cell Physiol. 2019. PMID: 30609027
-
Sensory plasticity of the carotid body: role of reactive oxygen species and physiological significance.Respir Physiol Neurobiol. 2011 Sep 30;178(3):375-80. doi: 10.1016/j.resp.2011.05.012. Epub 2011 May 18. Respir Physiol Neurobiol. 2011. PMID: 21621009 Free PMC article. Review.
-
Altered carotid body function by intermittent hypoxia in neonates and adults: relevance to recurrent apneas.Respir Physiol Neurobiol. 2007 Jul 1;157(1):148-53. doi: 10.1016/j.resp.2006.12.009. Epub 2007 Jan 11. Respir Physiol Neurobiol. 2007. PMID: 17317339 Review.
Cited by
-
The role of hypoxia-inducible factors in oxygen sensing by the carotid body.Adv Exp Med Biol. 2012;758:1-5. doi: 10.1007/978-94-007-4584-1_1. Adv Exp Med Biol. 2012. PMID: 23080136 Free PMC article. Review.
-
Regulation of carotid body oxygen sensing by hypoxia-inducible factors.Pflugers Arch. 2016 Jan;468(1):71-75. doi: 10.1007/s00424-015-1719-z. Epub 2015 Aug 13. Pflugers Arch. 2016. PMID: 26265380 Free PMC article. Review.
-
NADPH oxidase 2 mediates intermittent hypoxia-induced mitochondrial complex I inhibition: relevance to blood pressure changes in rats.Antioxid Redox Signal. 2011 Feb 15;14(4):533-42. doi: 10.1089/ars.2010.3213. Epub 2010 Oct 19. Antioxid Redox Signal. 2011. PMID: 20618070 Free PMC article.
-
Mechanisms of cardiovascular disease in obstructive sleep apnoea.J Thorac Dis. 2018 Dec;10(Suppl 34):S4201-S4211. doi: 10.21037/jtd.2018.08.56. J Thorac Dis. 2018. PMID: 30687536 Free PMC article. Review.
-
Anorexigenic Effects of Intermittent Hypoxia on the Gut-Brain Axis in Sleep Apnea Syndrome.Int J Mol Sci. 2021 Dec 29;23(1):364. doi: 10.3390/ijms23010364. Int J Mol Sci. 2021. PMID: 35008784 Free PMC article. Review.
References
-
- Baker TL, Fuller DD, Zabka AG, Mitchell GS. Respiratory plasticity: differential actions of continuous and episodic hypoxia and hypercapnia. Respir Physiol. 2001;129:25–35. - PubMed
-
- Bánfi B, Maturana A, Jaconi S, Arnaudeau S, Laforge T, Sinha B, Ligeti E, Demaurex N, Krause KH. A mammalian H+ channel generated through alternative splicing of the NADPH oxidase homolog NOH-1. Science. 2000;287:138–142. - PubMed
-
- Bedard K, Krause KH. The NOX family of ROS-generating NADPH oxidases: physiology and pathophysiology. Physiol Rev. 2007;87:245–313. - PubMed
-
- He L, Chen J, Dinger B, Sanders K, Sundar K, Hoidal J, Fidone S. Characteristics of carotid body chemosensitivity in NADPH oxidase-deficient mice. Am J Physiol Cell Physiol. 2002;282:C27–C33. - PubMed
Publication types
MeSH terms
Substances
Grants and funding
LinkOut - more resources
Full Text Sources
Other Literature Sources
Molecular Biology Databases
Miscellaneous