Redox-regulated chaperones
- PMID: 19368357
- PMCID: PMC2848813
- DOI: 10.1021/bi9003556
Redox-regulated chaperones
Abstract
Redox regulation of stress proteins, such as molecular chaperones, guarantees an immediate response to oxidative stress conditions. This review focuses on the two major classes of redox-regulated chaperones, Hsp33 in bacteria and typical 2-Cys peroxiredoxins in eukaryotes. Both proteins employ redox-sensitive cysteines, whose oxidation status directly controls their affinity for unfolding proteins and therefore their chaperone function. We will first discuss Hsp33, whose oxidative stress-induced disulfide bond formation triggers the partial unfolding of the chaperone, which, in turn, leads to the exposure of a high-affinity binding site for unfolded proteins. This rapid mode of activation makes Hsp33 essential for protecting bacteria against severe oxidative stress conditions, such as hypochlorite (i.e., bleach) treatment, which leads to widespread protein unfolding and aggregation. We will compare Hsp33 to the highly abundant eukaryotic typical 2-Cys peroxiredoxin, whose oxidative stress-induced sulfinic acid formation turns the peroxidase into a molecular chaperone in vitro and presumably in vivo. These examples illustrate how proteins use reversible cysteine modifications to rapidly adjust to oxidative stress conditions and demonstrate that redox regulation plays a vital role in protecting organisms against reactive oxygen species-mediated cell death.
Figures
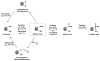
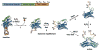
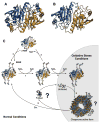
Similar articles
-
Activation of the redox-regulated chaperone Hsp33 by domain unfolding.J Biol Chem. 2004 May 7;279(19):20529-38. doi: 10.1074/jbc.M401764200. Epub 2004 Mar 15. J Biol Chem. 2004. PMID: 15023991
-
Unfolding of metastable linker region is at the core of Hsp33 activation as a redox-regulated chaperone.J Biol Chem. 2010 Apr 9;285(15):11243-51. doi: 10.1074/jbc.M109.084350. Epub 2010 Feb 5. J Biol Chem. 2010. PMID: 20139072 Free PMC article.
-
HSP33 in eukaryotes - an evolutionary tale of a chaperone adapted to photosynthetic organisms.Plant J. 2015 Jun;82(5):850-60. doi: 10.1111/tpj.12855. Plant J. 2015. PMID: 25892083
-
The redox switch that regulates molecular chaperones.Biomol Concepts. 2015 Aug;6(4):269-84. doi: 10.1515/bmc-2015-0015. Biomol Concepts. 2015. PMID: 26352357 Review.
-
Redox-regulated molecular chaperones.Cell Mol Life Sci. 2002 Oct;59(10):1624-31. doi: 10.1007/pl00012489. Cell Mol Life Sci. 2002. PMID: 12475172 Free PMC article. Review.
Cited by
-
Long-term survival of hydrated resting eggs from Brachionus plicatilis.PLoS One. 2012;7(1):e29365. doi: 10.1371/journal.pone.0029365. Epub 2012 Jan 9. PLoS One. 2012. PMID: 22253713 Free PMC article.
-
The Hsp60 protein of helicobacter pylori displays chaperone activity under acidic conditions.Biochem Biophys Rep. 2016 Nov 27;9:95-99. doi: 10.1016/j.bbrep.2016.11.011. eCollection 2017 Mar. Biochem Biophys Rep. 2016. PMID: 28955994 Free PMC article.
-
Moonlighting by different stressors: crystal structure of the chaperone species of a 2-Cys peroxiredoxin.Structure. 2012 Mar 7;20(3):429-39. doi: 10.1016/j.str.2012.01.004. Structure. 2012. PMID: 22405002 Free PMC article.
-
Human protein-disulfide isomerase is a redox-regulated chaperone activated by oxidation of domain a'.J Biol Chem. 2012 Jan 6;287(2):1139-49. doi: 10.1074/jbc.M111.303149. Epub 2011 Nov 16. J Biol Chem. 2012. PMID: 22090031 Free PMC article.
-
Insights into the Function of a Second, Nonclassical Ahp Peroxidase, AhpA, in Oxidative Stress Resistance in Bacillus subtilis.J Bacteriol. 2016 Jan 19;198(7):1044-57. doi: 10.1128/JB.00679-15. J Bacteriol. 2016. PMID: 26787766 Free PMC article.
References
-
- Schrader M, Fahimi HD. Peroxisomes and oxidative stress. Biochim Biophys Acta. 2006;1763:1755–1766. - PubMed
-
- Michiels C, Raes M, Toussaint O, Remacle J. Importance of Se-glutathione peroxidase, catalase, and Cu/Zn-SOD for cell survival against oxidative stress. Free Radical Biol Med. 1994;17:235–248. - PubMed
-
- D’Autreaux B, Toledano MB. ROS as signalling molecules: Mechanisms that generate specificity in ROS homeostasis. Nat Rev Mol Cell Biol. 2007;8:813–824. - PubMed
-
- Rhee SG. Cell signaling. H2O2, a necessary evil for cell signaling. Science. 2006;312:1882–1883. - PubMed
-
- Stadtman ER. Protein oxidation in aging and age-related diseases. Ann NY Acad Sci. 2001;928:22–38. - PubMed
Publication types
MeSH terms
Substances
Grants and funding
LinkOut - more resources
Full Text Sources