Fast adaptation and Ca2+ sensitivity of the mechanotransducer require myosin-XVa in inner but not outer cochlear hair cells
- PMID: 19339598
- PMCID: PMC2702482
- DOI: 10.1523/JNEUROSCI.4566-08.2009
Fast adaptation and Ca2+ sensitivity of the mechanotransducer require myosin-XVa in inner but not outer cochlear hair cells
Abstract
In inner ear hair cells, activation of mechanotransduction channels is followed by extremely rapid deactivation that depends on the influx of Ca(2+) through these channels. Although the molecular mechanisms of this "fast" adaptation are largely unknown, the predominant models assume Ca(2+) sensitivity as an intrinsic property of yet unidentified mechanotransduction channels. Here, we examined mechanotransduction in the hair cells of young postnatal shaker 2 mice (Myo15(sh2/sh2)). These mice have no functional myosin-XVa, which is critical for normal growth of mechanosensory stereocilia of hair cells. Although stereocilia of both inner and outer hair cells of Myo15(sh2/sh2) mice lack myosin-XVa and are abnormally short, these cells have dramatically different hair bundle morphology. Myo15(sh2/sh2) outer hair cells retain a staircase arrangement of the abnormally short stereocilia and prominent tip links. Myo15(sh2/sh2) inner hair cells do not have obliquely oriented tip links, and their mechanosensitivity is mediated exclusively by "top-to-top" links between equally short stereocilia. In both inner and outer hair cells of Myo15(sh2/sh2) mice, we found mechanotransduction responses with a normal "wild-type" amplitude and speed of activation. Surprisingly, only outer hair cells exhibit fast adaptation and sensitivity to extracellular Ca(2+). In Myo15(sh2/sh2) inner hair cells, fast adaptation is disrupted and the transduction current is insensitive to extracellular Ca(2+). We conclude that the Ca(2+) sensitivity of the mechanotransduction channels and the fast adaptation require a structural environment that is dependent on myosin-XVa and is disrupted in Myo15(sh2/sh2) inner hair cells, but not in Myo15(sh2/sh2) outer hair cells.
Figures
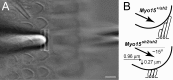
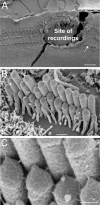
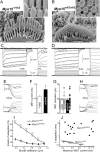
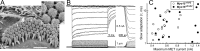
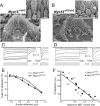
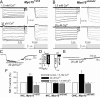
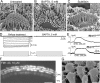
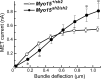
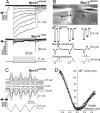
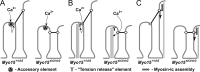
Similar articles
-
Myosin-XVa Controls Both Staircase Architecture and Diameter Gradation of Stereocilia Rows in the Auditory Hair Cell Bundles.J Assoc Res Otolaryngol. 2020 Apr;21(2):121-135. doi: 10.1007/s10162-020-00745-4. Epub 2020 Mar 9. J Assoc Res Otolaryngol. 2020. PMID: 32152769 Free PMC article.
-
Auditory mechanotransduction in the absence of functional myosin-XVa.J Physiol. 2006 Nov 1;576(Pt 3):801-8. doi: 10.1113/jphysiol.2006.118547. Epub 2006 Sep 14. J Physiol. 2006. PMID: 16973713 Free PMC article.
-
Whirler mutant hair cells have less severe pathology than shaker 2 or double mutants.J Assoc Res Otolaryngol. 2007 Sep;8(3):329-37. doi: 10.1007/s10162-007-0083-x. Epub 2007 Jul 6. J Assoc Res Otolaryngol. 2007. PMID: 17619105 Free PMC article.
-
Tonotopy in calcium homeostasis and vulnerability of cochlear hair cells.Hear Res. 2019 May;376:11-21. doi: 10.1016/j.heares.2018.11.002. Epub 2018 Nov 16. Hear Res. 2019. PMID: 30473131 Free PMC article. Review.
-
Myosin I and adaptation of mechanical transduction by the inner ear.Philos Trans R Soc Lond B Biol Sci. 2004 Dec 29;359(1452):1945-51. doi: 10.1098/rstb.2004.1564. Philos Trans R Soc Lond B Biol Sci. 2004. PMID: 15647170 Free PMC article. Review.
Cited by
-
Sensing sound: molecules that orchestrate mechanotransduction by hair cells.Trends Neurosci. 2012 Apr;35(4):220-9. doi: 10.1016/j.tins.2011.10.007. Epub 2011 Dec 15. Trends Neurosci. 2012. PMID: 22177415 Free PMC article. Review.
-
Is TMC1 the Hair Cell Mechanotransducer Channel?Biophys J. 2016 Jul 12;111(1):3-9. doi: 10.1016/j.bpj.2016.05.032. Biophys J. 2016. PMID: 27410728 Free PMC article. Review.
-
Study of the Mechanisms by Which Aminoglycoside Damage Is Prevented in Chick Embryonic Hair Cells.J Assoc Res Otolaryngol. 2019 Feb;20(1):21-35. doi: 10.1007/s10162-018-00700-4. Epub 2018 Oct 18. J Assoc Res Otolaryngol. 2019. PMID: 30341698 Free PMC article.
-
Eps8 regulates hair bundle length and functional maturation of mammalian auditory hair cells.PLoS Biol. 2011 Apr;9(4):e1001048. doi: 10.1371/journal.pbio.1001048. Epub 2011 Apr 19. PLoS Biol. 2011. PMID: 21526224 Free PMC article.
-
Integrating the biophysical and molecular mechanisms of auditory hair cell mechanotransduction.Nat Commun. 2011 Nov 1;2:523. doi: 10.1038/ncomms1533. Nat Commun. 2011. PMID: 22045002 Free PMC article. Review.
References
-
- Ahmed ZM, Goodyear R, Riazuddin S, Lagziel A, Legan PK, Behra M, Burgess SM, Lilley KS, Wilcox ER, Riazuddin S, Griffith AJ, Frolenkov GI, Belyantseva IA, Richardson GP, Friedman TB. The tip-link antigen, a protein associated with the transduction complex of sensory hair cells, is protocadherin-15. J Neurosci. 2006;26:7022–7034. - PMC - PubMed
-
- Assad JA, Shepherd GM, Corey DP. Tip-link integrity and mechanical transduction in vertebrate hair cells. Neuron. 1991;7:985–994. - PubMed
Publication types
MeSH terms
Substances
Grants and funding
LinkOut - more resources
Full Text Sources
Molecular Biology Databases
Miscellaneous