Mrpl36 is important for generation of assembly competent proteins during mitochondrial translation
- PMID: 19339279
- PMCID: PMC2682602
- DOI: 10.1091/mbc.e08-12-1162
Mrpl36 is important for generation of assembly competent proteins during mitochondrial translation
Abstract
The complexes of the respiratory chain represent mosaics of nuclear and mitochondrially encoded components. The processes by which synthesis and assembly of the various subunits are coordinated remain largely elusive. During evolution, many proteins of the mitochondrial ribosome acquired additional domains pointing at specific properties or functions of the translation machinery in mitochondria. Here, we analyzed the function of Mrpl36, a protein associated with the large subunit of the mitochondrial ribosome. This protein, homologous to the ribosomal protein L31 from bacteria, contains a mitochondria-specific C-terminal domain that is not required for protein synthesis per se; however, its absence decreases stability of Mrpl36. Cells lacking this C-terminal domain can still synthesize proteins, but these translation products fail to be properly assembled into respiratory chain complexes and are rapidly degraded. Surprisingly, overexpression of Mrpl36 seems to even increase the efficiency of mitochondrial translation. Our data suggest that Mrpl36 plays a critical role during translation that determines the rate of respiratory chain assembly. This important function seems to be carried out by a stabilizing activity of Mrpl36 on the interaction between large and small ribosomal subunits, which could influence accuracy of protein synthesis.
Figures
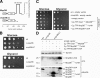
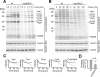
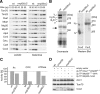
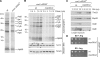
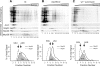
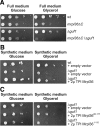
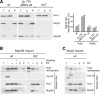
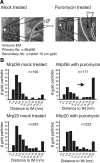
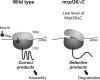
Similar articles
-
MrpL36p, a highly diverged L31 ribosomal protein homolog with additional functional domains in Saccharomyces cerevisiae mitochondria.Genetics. 2004 May;167(1):65-75. doi: 10.1534/genetics.167.1.65. Genetics. 2004. PMID: 15166137 Free PMC article.
-
Mapping of the Saccharomyces cerevisiae Oxa1-mitochondrial ribosome interface and identification of MrpL40, a ribosomal protein in close proximity to Oxa1 and critical for oxidative phosphorylation complex assembly.Eukaryot Cell. 2009 Nov;8(11):1792-802. doi: 10.1128/EC.00219-09. Epub 2009 Sep 25. Eukaryot Cell. 2009. PMID: 19783770 Free PMC article.
-
The C-terminal extension domain of Saccharomyces cerevisiae MrpL32, a homolog of ribosomal protein L32, functions in trans to support mitochondrial translation.Genes Genet Syst. 2018 Jul 13;93(1):21-24. doi: 10.1266/ggs.17-00023. Epub 2018 Jan 17. Genes Genet Syst. 2018. PMID: 29343666
-
The polypeptide tunnel exit of the mitochondrial ribosome is tailored to meet the specific requirements of the organelle.Bioessays. 2010 Dec;32(12):1050-7. doi: 10.1002/bies.201000081. Epub 2010 Oct 21. Bioessays. 2010. PMID: 20967780 Review.
-
Clingy genes: Why were genes for ribosomal proteins retained in many mitochondrial genomes?Biochim Biophys Acta Bioenerg. 2020 Nov 1;1861(11):148275. doi: 10.1016/j.bbabio.2020.148275. Epub 2020 Jul 23. Biochim Biophys Acta Bioenerg. 2020. PMID: 32712152 Review.
Cited by
-
Ribosome-binding proteins Mdm38 and Mba1 display overlapping functions for regulation of mitochondrial translation.Mol Biol Cell. 2010 Jun 15;21(12):1937-44. doi: 10.1091/mbc.e10-02-0101. Epub 2010 Apr 28. Mol Biol Cell. 2010. PMID: 20427570 Free PMC article.
-
Integrated analysis of the critical region 5p15.3-p15.2 associated with cri-du-chat syndrome.Genet Mol Biol. 2019;42(1 suppl 1):186-196. doi: 10.1590/1678-4685-GMB-2018-0173. Epub 2019 Apr 11. Genet Mol Biol. 2019. PMID: 30985858 Free PMC article.
-
Dichloroacetate reverses the hypoxic adaptation to bevacizumab and enhances its antitumor effects in mouse xenografts.J Mol Med (Berl). 2013 Jun;91(6):749-58. doi: 10.1007/s00109-013-0996-2. Epub 2013 Jan 30. J Mol Med (Berl). 2013. PMID: 23361368 Free PMC article.
-
Effects of physical exercise on muscle metabolism and meat quality characteristics of Mongolian sheep.Food Sci Nutr. 2022 Mar 1;10(5):1494-1509. doi: 10.1002/fsn3.2768. eCollection 2022 May. Food Sci Nutr. 2022. PMID: 35592278 Free PMC article.
-
Multi-omic integration by machine learning (MIMaL).Bioinformatics. 2022 Oct 31;38(21):4908-4918. doi: 10.1093/bioinformatics/btac631. Bioinformatics. 2022. PMID: 36106996 Free PMC article.
References
-
- Ackerman S. H., Tzagoloff A. Function, structure, and biogenesis of mitochondrial ATP synthase. Prog. Nucleic Acid Res. Mol. Biol. 2005;80:95–133. - PubMed
-
- Bauerschmitt H., Funes S., Herrmann J. M. The membrane-bound GTPase Guf1 promotes mitochondrial protein synthesis under suboptimal conditions. J. Biol. Chem. 2008;283:17139–17146. - PubMed
Publication types
MeSH terms
Substances
LinkOut - more resources
Full Text Sources
Molecular Biology Databases