A role of DNA-PK for the metabolic gene regulation in response to insulin
- PMID: 19303849
- PMCID: PMC2768498
- DOI: 10.1016/j.cell.2008.12.040
A role of DNA-PK for the metabolic gene regulation in response to insulin
Abstract
Fatty acid synthase (FAS) is a central enzyme in lipogenesis and transcriptionally activated in response to feeding and insulin signaling. The transcription factor USF is required for the activation of FAS transcription, and we show here that USF phosphorylation by DNA-PK, which is dephosphorylated by PP1 in response to feeding, triggers a switch-like mechanism. Under fasting conditions, USF-1 is deacetylated by HDAC9, causing promoter inactivation. In contrast, feeding induces the recruitment of DNA-PK to USF-1 and its phosphorylation, which then allows recruitment of P/CAF, resulting in USF-1 acetylation and FAS promoter activation. DNA break/repair components associated with USF induce transient DNA breaks during FAS activation. In DNA-PK-deficient SCID mice, feeding-induced USF-1 phosphorylation/acetylation, DNA breaks, and FAS activation leading to lipogenesis are impaired, resulting in decreased triglyceride levels. Our study demonstrates that a kinase central to the DNA damage response mediates metabolic gene activation.
Figures
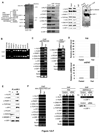
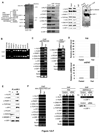
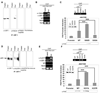
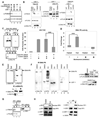
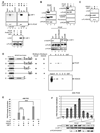
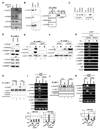
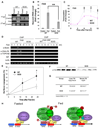
Similar articles
-
Phosphorylation and recruitment of BAF60c in chromatin remodeling for lipogenesis in response to insulin.Mol Cell. 2013 Jan 24;49(2):283-97. doi: 10.1016/j.molcel.2012.10.028. Epub 2012 Dec 6. Mol Cell. 2013. PMID: 23219531 Free PMC article.
-
DNA-PK: relaying the insulin signal to USF in lipogenesis.Cell Cycle. 2009 Jul 1;8(13):1977-8. doi: 10.4161/cc.8.13.8941. Epub 2009 Jul 15. Cell Cycle. 2009. PMID: 19550139 Free PMC article. No abstract available.
-
Direct interaction between USF and SREBP-1c mediates synergistic activation of the fatty-acid synthase promoter.J Biol Chem. 2007 Feb 23;282(8):5453-67. doi: 10.1074/jbc.M610566200. Epub 2006 Dec 31. J Biol Chem. 2007. PMID: 17197698
-
Insulin regulation of fatty acid synthase gene transcription: roles of USF and SREBP-1c.IUBMB Life. 2004 Oct;56(10):595-600. doi: 10.1080/15216540400022474. IUBMB Life. 2004. PMID: 15814457 Review.
-
[From the glycogenic function of the liver to gene regulation by glucose].C R Seances Soc Biol Fil. 1998;192(5):813-27. C R Seances Soc Biol Fil. 1998. PMID: 9871795 Review. French.
Cited by
-
Histone deacetylase 9 (HDAC9) regulates the functions of the ATDC (TRIM29) protein.J Biol Chem. 2010 Dec 10;285(50):39329-38. doi: 10.1074/jbc.M110.179333. Epub 2010 Oct 14. J Biol Chem. 2010. PMID: 20947501 Free PMC article.
-
Diet and Genetics Influence Beef Cattle Performance and Meat Quality Characteristics.Foods. 2019 Dec 6;8(12):648. doi: 10.3390/foods8120648. Foods. 2019. PMID: 31817572 Free PMC article. Review.
-
Overexpression of CLC-3 is regulated by XRCC5 and is a poor prognostic biomarker for gastric cancer.J Hematol Oncol. 2018 Sep 14;11(1):115. doi: 10.1186/s13045-018-0660-y. J Hematol Oncol. 2018. PMID: 30217218 Free PMC article.
-
Histone deacetylase 9 deficiency protects against effector T cell-mediated systemic autoimmunity.J Biol Chem. 2011 Aug 19;286(33):28833-28843. doi: 10.1074/jbc.M111.233932. Epub 2011 Jun 27. J Biol Chem. 2011. PMID: 21708950 Free PMC article.
-
Comparative analysis of basal and etoposide-induced alterations in gene expression by DNA-PKcs kinase activity.Front Genet. 2024 Mar 21;15:1276365. doi: 10.3389/fgene.2024.1276365. eCollection 2024. Front Genet. 2024. PMID: 38577247 Free PMC article.
References
-
- Allen PB, Kwon YG, Nairn AC, Greengard P. Isolation and characterization of PNUTS, a putative protein phosphatase 1 nuclear targeting subunit. J. Biol. Chem. 1998;273:4089–4095. - PubMed
-
- Brady MJ, Saltiel AR. The role of protein phosphatase-1 in insulin action. Recent Prog. Horm. Res. 2001;56:157–173. - PubMed
-
- Casado M, Vallet VS, Kahn A, Vaulont S. Essential role in vivo of upstream stimulatory factors for a normal dietary response of the fatty acid synthase gene in the liver. J. Biol. Chem. 1999;274:2009–2013. - PubMed
-
- Collis SJ, DeWeese TL, Jeggo PA, Parker AR. The life and death of DNA-PK. Oncogene. 2005;24:949–961. - PubMed
-
- Corre S, Galibert MD. Upstream stimulating factors: highly versatile stress-responsive transcription factors. Pigment Cell Res. 2005;18:337–348. - PubMed
Publication types
MeSH terms
Substances
Grants and funding
LinkOut - more resources
Full Text Sources
Other Literature Sources
Medical
Molecular Biology Databases
Research Materials
Miscellaneous