Intracellular trafficking of presenilin 1 is regulated by beta-amyloid precursor protein and phospholipase D1
- PMID: 19276086
- PMCID: PMC2673283
- DOI: 10.1074/jbc.M808497200
Intracellular trafficking of presenilin 1 is regulated by beta-amyloid precursor protein and phospholipase D1
Abstract
Excessive accumulation of beta-amyloid peptides in the brain is a major cause for the pathogenesis of Alzheimer disease. beta-Amyloid is derived from beta-amyloid precursor protein (APP) through sequential cleavages by beta- and gamma-secretases, whose enzymatic activities are tightly controlled by subcellular localization. Delineation of how intracellular trafficking of these secretases and APP is regulated is important for understanding Alzheimer disease pathogenesis. Although APP trafficking is regulated by multiple factors including presenilin 1 (PS1), a major component of the gamma-secretase complex, and phospholipase D1 (PLD1), a phospholipid-modifying enzyme, regulation of intracellular trafficking of PS1/gamma-secretase and beta-secretase is less clear. Here we demonstrate that APP can reciprocally regulate PS1 trafficking; APP deficiency results in faster transport of PS1 from the trans-Golgi network to the cell surface and increased steady state levels of PS1 at the cell surface, which can be reversed by restoring APP levels. Restoration of APP in APP-deficient cells also reduces steady state levels of other gamma-secretase components (nicastrin, APH-1, and PEN-2) and the cleavage of Notch by PS1/gamma-secretase that is more highly correlated with cell surface levels of PS1 than with APP overexpression levels, supporting the notion that Notch is mainly cleaved at the cell surface. In contrast, intracellular trafficking of beta-secretase (BACE1) is not regulated by APP. Moreover, we find that PLD1 also regulates PS1 trafficking and that PLD1 overexpression promotes cell surface accumulation of PS1 in an APP-independent manner. Our results clearly elucidate a physiological function of APP in regulating protein trafficking and suggest that intracellular trafficking of PS1/gamma-secretase is regulated by multiple factors, including APP and PLD1.
Figures
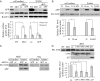
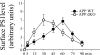
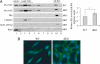
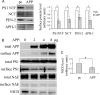
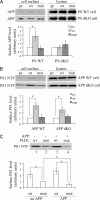
Similar articles
-
Nicastrin is critical for stability and trafficking but not association of other presenilin/gamma-secretase components.J Biol Chem. 2005 Apr 29;280(17):17020-6. doi: 10.1074/jbc.M409467200. Epub 2005 Feb 11. J Biol Chem. 2005. PMID: 15711015 Free PMC article.
-
Effects of Folic Acid on Secretases Involved in Aβ Deposition in APP/PS1 Mice.Nutrients. 2016 Sep 9;8(9):556. doi: 10.3390/nu8090556. Nutrients. 2016. PMID: 27618097 Free PMC article.
-
Nicastrin is required for assembly of presenilin/gamma-secretase complexes to mediate Notch signaling and for processing and trafficking of beta-amyloid precursor protein in mammals.J Neurosci. 2003 Apr 15;23(8):3272-7. doi: 10.1523/JNEUROSCI.23-08-03272.2003. J Neurosci. 2003. PMID: 12716934 Free PMC article.
-
The role of membrane trafficking in the processing of amyloid precursor protein and production of amyloid peptides in Alzheimer's disease.Biochim Biophys Acta Biomembr. 2019 Apr 1;1861(4):697-712. doi: 10.1016/j.bbamem.2018.11.013. Epub 2019 Jan 11. Biochim Biophys Acta Biomembr. 2019. PMID: 30639513 Review.
-
Role of presenilin in gamma-secretase cleavage of amyloid precursor protein.Exp Gerontol. 2000 Jul;35(4):453-60. doi: 10.1016/s0531-5565(00)00111-x. Exp Gerontol. 2000. PMID: 10959033 Review.
Cited by
-
Novel susceptibility loci for Alzheimer's disease.Future Neurol. 2015 Dec;10(6):547-558. doi: 10.2217/fnl.15.42. Future Neurol. 2015. PMID: 27057151 Free PMC article.
-
APP processing in Alzheimer's disease.Mol Brain. 2011 Jan 7;4:3. doi: 10.1186/1756-6606-4-3. Mol Brain. 2011. PMID: 21214928 Free PMC article. Review.
-
Linking lipids to Alzheimer's disease: cholesterol and beyond.Nat Rev Neurosci. 2011 May;12(5):284-96. doi: 10.1038/nrn3012. Epub 2011 Mar 30. Nat Rev Neurosci. 2011. PMID: 21448224 Free PMC article. Review.
-
Alzheimer amyloid beta inhibition of Eg5/kinesin 5 reduces neurotrophin and/or transmitter receptor function.Neurobiol Aging. 2014 Aug;35(8):1839-49. doi: 10.1016/j.neurobiolaging.2014.02.006. Epub 2014 Feb 10. Neurobiol Aging. 2014. PMID: 24636920 Free PMC article.
-
Altered expression of claudin family proteins in Alzheimer's disease and vascular dementia brains.J Cell Mol Med. 2010 May;14(5):1088-100. doi: 10.1111/j.1582-4934.2009.00999.x. J Cell Mol Med. 2010. PMID: 20041969 Free PMC article.
References
-
- Selkoe, D. J. (1998) Trends Cell Biol. 8 447-453 - PubMed
-
- Zhang, Y. W., and Xu, H. (2007) Curr. Mol. Med. 7 687-696 - PubMed
-
- Vassar, R., Bennett, B. D., Babu-Khan, S., Kahn, S., Mendiaz, E. A., Denis, P., Teplow, D. B., Ross, S., Amarante, P., Loeloff, R., Luo, Y., Fisher, S., Fuller, J., Edenson, S., Lile, J., Jarosinski, M. A., Biere, A. L., Curran, E., Burgess, T., Louis, J. C., Collins, F., Treanor, J., Rogers, G., and Citron, M. (1999) Science 286 735-741 - PubMed
-
- Sinha, S., Anderson, J. P., Barbour, R., Basi, G. S., Caccavello, R., Davis, D., Doan, M., Dovey, H. F., Frigon, N., Hong, J., Jacobson-Croak, K., Jewett, N., Keim, P., Knops, J., Lieberburg, I., Power, M., Tan, H., Tatsuno, G., Tung, J., Schenk, D., Seubert, P., Suomensaari, S. M., Wang, S., Walker, D., Zhao, J., McConlogue, L., and John, V. (1999) Nature 402 537-540 - PubMed
Publication types
MeSH terms
Substances
Grants and funding
LinkOut - more resources
Full Text Sources