The why and how of DNA unlinking
- PMID: 19240147
- PMCID: PMC2647305
- DOI: 10.1093/nar/gkp041
The why and how of DNA unlinking
Abstract
The nucleotide sequence of DNA is the repository of hereditary information. Yet, it is now clear that the DNA itself plays an active role in regulating the ability of the cell to extract its information. Basic biological processes, including control of gene transcription, faithful DNA replication and segregation, maintenance of the genome and cellular differentiation are subject to the conformational and topological properties of DNA in addition to the regulation imparted by the sequence itself. How do these DNA features manifest such striking effects and how does the cell regulate them? In this review, we describe how misregulation of DNA topology can lead to cellular dysfunction. We then address how cells prevent these topological problems. We close with a discussion on recent theoretical advances indicating that the topological problems, themselves, can provide the cues necessary for their resolution by type-2 topoisomerases.
Figures
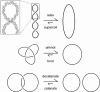
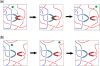
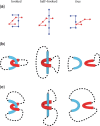
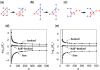
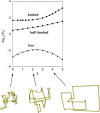
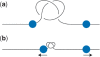
Similar articles
-
Unlinking of supercoiled DNA catenanes by type IIA topoisomerases.Biophys J. 2011 Sep 21;101(6):1403-11. doi: 10.1016/j.bpj.2011.08.011. Epub 2011 Sep 20. Biophys J. 2011. PMID: 21943421 Free PMC article.
-
Simulation of DNA catenanes.Phys Chem Chem Phys. 2009 Dec 7;11(45):10543-52. doi: 10.1039/b910812b. Epub 2009 Oct 23. Phys Chem Chem Phys. 2009. PMID: 20145800 Free PMC article. Review.
-
DNA disentangling by type-2 topoisomerases.J Mol Biol. 2004 Jul 23;340(5):933-9. doi: 10.1016/j.jmb.2004.05.034. J Mol Biol. 2004. PMID: 15236957
-
Single-molecule study of DNA unlinking by eukaryotic and prokaryotic type-II topoisomerases.Proc Natl Acad Sci U S A. 2003 Aug 19;100(17):9820-5. doi: 10.1073/pnas.1631550100. Epub 2003 Aug 5. Proc Natl Acad Sci U S A. 2003. PMID: 12902541 Free PMC article.
-
DNA-Topology Simplification by Topoisomerases.Molecules. 2021 Jun 3;26(11):3375. doi: 10.3390/molecules26113375. Molecules. 2021. PMID: 34204901 Free PMC article. Review.
Cited by
-
Phytochemicals as Anticancer and Chemopreventive Topoisomerase II Poisons.Phytochem Rev. 2014 Mar 1;13(1):19-35. doi: 10.1007/s11101-013-9291-7. Phytochem Rev. 2014. PMID: 24678287 Free PMC article.
-
Direct measurement of DNA bending by type IIA topoisomerases: implications for non-equilibrium topology simplification.Nucleic Acids Res. 2011 Jul;39(13):5729-43. doi: 10.1093/nar/gkr109. Epub 2011 Mar 17. Nucleic Acids Res. 2011. PMID: 21421557 Free PMC article.
-
Two-Dimensional Gel Electrophoresis to Resolve DNA Topoisomers.Methods Mol Biol. 2020;2119:15-24. doi: 10.1007/978-1-0716-0323-9_2. Methods Mol Biol. 2020. PMID: 31989511 Free PMC article.
-
Basis for the discrimination of supercoil handedness during DNA cleavage by human and bacterial type II topoisomerases.Nucleic Acids Res. 2023 May 8;51(8):3888-3902. doi: 10.1093/nar/gkad190. Nucleic Acids Res. 2023. PMID: 36999602 Free PMC article.
-
Insights into Genome Architecture Deduced from the Properties of Short Lac Repressor-mediated DNA Loops.Biophys Rev. 2016 Nov;8(1 Suppl):135-144. doi: 10.1007/s12551-016-0209-7. Epub 2016 Jul 2. Biophys Rev. 2016. PMID: 28133491 Free PMC article.
References
-
- Watson JD, Crick F.HC. Molecular structure of nucleic acids: a structure for deoxyribose nucleic acid. Nature. 1953;171:737–738. - PubMed
-
- Schoeffler AJ, Berger JM. DNA topoisomerases: harnessing and constraining energy to govern chromosome topology. Q. Rev. Biophys. 2008;41:41–101. - PubMed
-
- Fogg JM, Catanese DJ, Randall GL, Swick MC, Zechiedrich L. Differences between positively and negatively supercoiled DNA that topoisomerases may distinguish. Proc. Institute Math. Appl. 2008 (in press)
-
- Zechiedrich EL, Khodursky AB, Bachellier S, Schneider R, Chen D, Lilley D.MJ, Cozzarelli NR. Roles of topoisomerases in maintaining steady-state DNA supercoiling in Escherichia coli. J. Biol. Chem. 2000;275:8103–8113. - PubMed
-
- Travers A, Muskhelishvili G. DNA supercoiling - a global transcriptional regulator for enterobacterial growth? Nat. Rev. Microbiol. 2005;3:157–169. - PubMed