A conditional transposon-based insertional mutagenesis screen for genes associated with mouse hepatocellular carcinoma
- PMID: 19234449
- PMCID: PMC2712727
- DOI: 10.1038/nbt.1526
A conditional transposon-based insertional mutagenesis screen for genes associated with mouse hepatocellular carcinoma
Abstract
We describe a system that permits conditional mobilization of a Sleeping Beauty (SB) transposase allele by Cre recombinase to induce cancer specifically in a tissue of interest. To demonstrate its potential for developing tissue-specific models of cancer in mice, we limit SB transposition to the liver by placing Cre expression under the control of an albumin enhancer/promoter sequence and screen for hepatocellular carcinoma (HCC)-associated genes. From 8,060 nonredundant insertions cloned from 68 tumor nodules and comparative analysis with data from human HCC samples, we identify 19 loci strongly implicated in causing HCC. These encode genes, such as EGFR and MET, previously associated with HCC and others, such as UBE2H, that are potential new targets for treating this neoplasm. Our system, which could be modified to drive transposon-based insertional mutagenesis wherever tissue-specific Cre expression is possible, promises to enhance understanding of cancer genomes and identify new targets for therapeutic development.
Figures
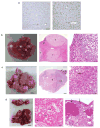
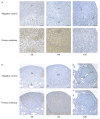
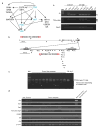
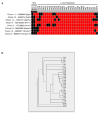
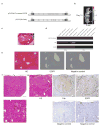
Comment in
-
When sleeping beauty wakes up and jumps into a cancer-minded environment.Hepatology. 2009 Jun;49(6):2122-4. doi: 10.1002/hep.23030. Hepatology. 2009. PMID: 19479787 No abstract available.
Similar articles
-
Identification of Sleeping Beauty transposon insertions in solid tumors using linker-mediated PCR.J Vis Exp. 2013 Feb 1;(72):e50156. doi: 10.3791/50156. J Vis Exp. 2013. PMID: 23407503 Free PMC article.
-
A modified sleeping beauty transposon system that can be used to model a wide variety of human cancers in mice.Cancer Res. 2009 Oct 15;69(20):8150-6. doi: 10.1158/0008-5472.CAN-09-1135. Epub 2009 Oct 6. Cancer Res. 2009. PMID: 19808965 Free PMC article.
-
Sleeping Beauty Insertional Mutagenesis in Mice Identifies Drivers of Steatosis-Associated Hepatic Tumors.Cancer Res. 2017 Dec 1;77(23):6576-6588. doi: 10.1158/0008-5472.CAN-17-2281. Epub 2017 Oct 9. Cancer Res. 2017. PMID: 28993411 Free PMC article.
-
[Advances in studies of Sleeping Beauty transposon].Yi Chuan. 2007 Jul;29(7):785-92. doi: 10.1360/yc-007-0785. Yi Chuan. 2007. PMID: 17646142 Review. Chinese.
-
Sleeping beauty--a mouse model for all cancers?Cancer Lett. 2012 Apr 1;317(1):1-8. doi: 10.1016/j.canlet.2011.11.006. Epub 2011 Nov 10. Cancer Lett. 2012. PMID: 22079740 Review.
Cited by
-
Genetically engineered mouse models of PI3K signaling in breast cancer.Mol Oncol. 2013 Apr;7(2):146-64. doi: 10.1016/j.molonc.2013.02.003. Epub 2013 Feb 11. Mol Oncol. 2013. PMID: 23478237 Free PMC article. Review.
-
Controlled insertional mutagenesis using a LINE-1 (ORFeus) gene-trap mouse model.Proc Natl Acad Sci U S A. 2013 Jul 16;110(29):E2706-13. doi: 10.1073/pnas.1302504110. Epub 2013 Jul 1. Proc Natl Acad Sci U S A. 2013. PMID: 23818630 Free PMC article.
-
The deubiquitinase USP9X suppresses pancreatic ductal adenocarcinoma.Nature. 2012 Apr 29;486(7402):266-70. doi: 10.1038/nature11114. Nature. 2012. PMID: 22699621 Free PMC article.
-
Cancer gene discovery in hepatocellular carcinoma.J Hepatol. 2010 Jun;52(6):921-9. doi: 10.1016/j.jhep.2009.12.034. Epub 2010 Mar 20. J Hepatol. 2010. PMID: 20385424 Free PMC article. Review.
-
Liver regeneration after partial hepatectomy: critical analysis of mechanistic dilemmas.Am J Pathol. 2010 Jan;176(1):2-13. doi: 10.2353/ajpath.2010.090675. Epub 2009 Dec 17. Am J Pathol. 2010. PMID: 20019184 Free PMC article. Review.
References
-
- Bellen HJ, et al. P-element-mediated enhancer detection: a versatile method to study development in Drosophila. Genes Dev. 1989;3:1288–1300. - PubMed
-
- Ivics Z, Hackett PB, Plasterk RH, Izsvak Z. Molecular reconstruction of Sleeping Beauty, a Tc1-like transposon from fish, and its transposition in human cells. Cell. 1997;91:501–510. - PubMed
-
- Dupuy AJ, Fritz S, Largaespada DA. Transposition and gene disruption in the male germline of the mouse. Genesis. 2001;30:82–88. - PubMed
Publication types
MeSH terms
Substances
Grants and funding
LinkOut - more resources
Full Text Sources
Other Literature Sources
Molecular Biology Databases
Research Materials
Miscellaneous