Determinants of water permeability through nanoscopic hydrophilic channels
- PMID: 19186131
- PMCID: PMC2716641
- DOI: 10.1016/j.bpj.2008.09.059
Determinants of water permeability through nanoscopic hydrophilic channels
Abstract
Naturally occurring pores show a variety of polarities and sizes that are presumably directly linked to their biological function. Many biological channels are selective toward permeants similar or smaller in size than water molecules, and therefore their pores operate in the regime of single-file water pores. Intrinsic factors affecting water permeability through such pores include the channel-membrane match, the structural stability of the channel, the channel geometry and channel-water affinity. We present an extensive molecular dynamics study on the role of the channel geometry and polarity on the water osmotic and diffusive permeability coefficients. We show that the polarity of the naturally occurring peptidic channels is close to optimal for water permeation, and that the water mobility for a wide range of channel polarities is essentially length independent. By systematically varying the geometry and polarity of model hydrophilic pores, based on the fold of gramicidin A, the water density, occupancy, and permeability are studied. Our focus is on the characterization of the transition between different permeation regimes in terms of the structure of water in the pores, the average pore occupancy and the dynamics of the permeating water molecules. We show that a general relationship between osmotic and diffusive water permeability coefficients in the single-file regime accounts for the time averaged pore occupancy, and that the dynamics of the permeating water molecules through narrow non single file channels effectively behaves like independent single-file columns.
Figures
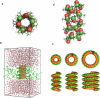
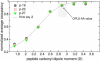
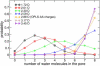
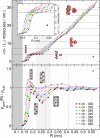
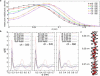
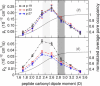
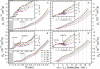
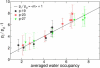
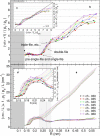
Similar articles
-
Water permeability of gramicidin A-treated lipid bilayer membranes.J Gen Physiol. 1978 Sep;72(3):341-50. doi: 10.1085/jgp.72.3.341. J Gen Physiol. 1978. PMID: 81265 Free PMC article.
-
The role of the dielectric barrier in narrow biological channels: a novel composite approach to modeling single-channel currents.Biophys J. 2003 Jun;84(6):3646-61. doi: 10.1016/S0006-3495(03)75095-4. Biophys J. 2003. PMID: 12770873 Free PMC article.
-
Invariance of single-file water mobility in gramicidin-like peptidic pores as function of pore length.Biophys J. 2007 Jun 1;92(11):3930-7. doi: 10.1529/biophysj.106.102921. Epub 2007 Mar 16. Biophys J. 2007. PMID: 17369423 Free PMC article.
-
Emerging issues of connexin channels: biophysics fills the gap.Q Rev Biophys. 2001 Aug;34(3):325-472. doi: 10.1017/s0033583501003705. Q Rev Biophys. 2001. PMID: 11838236 Review.
-
Water in ion channels and pores--simulation studies.Novartis Found Symp. 2002;245:66-78; discussion 79-83, 165-8. Novartis Found Symp. 2002. PMID: 12027016 Review.
Cited by
-
Teaching old coefficients new tricks: new insight into the meaning of the osmotic and diffusive permeation coefficients.Biophys J. 2009 Feb;96(3):763-4. doi: 10.1016/j.bpj.2008.10.048. Biophys J. 2009. PMID: 19186119 Free PMC article. No abstract available.
-
Water Determines the Structure and Dynamics of Proteins.Chem Rev. 2016 Jul 13;116(13):7673-97. doi: 10.1021/acs.chemrev.5b00664. Epub 2016 May 17. Chem Rev. 2016. PMID: 27186992 Free PMC article. Review.
-
A hard sphere model for single-file water transport across biological membranes.Eur Phys J E Soft Matter. 2024 Apr 15;47(4):27. doi: 10.1140/epje/s10189-024-00419-6. Eur Phys J E Soft Matter. 2024. PMID: 38619676 Free PMC article.
-
Water Transport through Nanotubes with Varying Interaction Strength between Tube Wall and Water.J Phys Chem Lett. 2011 Oct 28;2(23):2978-2983. doi: 10.1021/jz2012319. J Phys Chem Lett. 2011. PMID: 25606067 Free PMC article.
-
Tutorial for Stopped-Flow Water Flux Measurements: Why a Report about "Ultrafast Water Permeation through Nanochannels with a Densely Fluorous Interior Surface" Is Flawed.Biomolecules. 2023 Feb 24;13(3):431. doi: 10.3390/biom13030431. Biomolecules. 2023. PMID: 36979366 Free PMC article.
References
-
- Agre P. Aquaporin water channels (Nobel Lecture) Angew. Chem. Int. Ed. 2004;43:4278–4290. - PubMed
-
- Jensen, P.H., and D. Keller, 2006. Membrane for filtering of water. Patent number WO 2006/122566.
-
- Cornell B.A., Braach-Maksvytis V.L., King L.G., Osman P.D., Raguse B. A biosensor that uses ion-channel switches. Nature. 1997;387:580–583. - PubMed
-
- Hirano A., Wakabayashi M., Matsuno Y., Sugawara M. A single-channel sensor based on gramicidin controlled by molecular recognition at bilayer lipid membranes containing receptor. Biosens. Bioelectron. 2003;18:973–983. - PubMed
-
- Futaki S., Zhang Y.J., Kiwada T., Nakase I., Yagami T. Gramicidin-based channel systems for the detection of protein-ligand interaction. Bioorg. Med. Chem. 2004;12:1343–1350. - PubMed
Publication types
MeSH terms
Substances
LinkOut - more resources
Full Text Sources
Other Literature Sources