Walking the walk: how kinesin and dynein coordinate their steps
- PMID: 19179063
- PMCID: PMC2668149
- DOI: 10.1016/j.ceb.2008.12.002
Walking the walk: how kinesin and dynein coordinate their steps
Abstract
Molecular motors drive key biological processes such as cell division, intracellular organelle transport, and sperm propulsion and defects in motor function can give rise to various human diseases. Two dimeric microtubule-based motor proteins, kinesin-1 and cytoplasmic dynein can take over one hundred steps without detaching from the track. In this review, we discuss how these processive motors coordinate the activities of their two identical motor domains so that they can walk along microtubules.
Figures
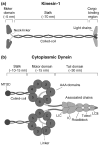
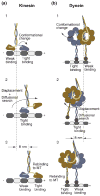
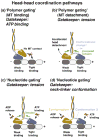
Similar articles
-
Kinesin-1, -2, and -3 motors use family-specific mechanochemical strategies to effectively compete with dynein during bidirectional transport.Elife. 2022 Sep 20;11:e82228. doi: 10.7554/eLife.82228. Elife. 2022. PMID: 36125250 Free PMC article.
-
Intraflagellar transport dynein is autoinhibited by trapping of its mechanical and track-binding elements.Nat Struct Mol Biol. 2017 May;24(5):461-468. doi: 10.1038/nsmb.3391. Epub 2017 Apr 10. Nat Struct Mol Biol. 2017. PMID: 28394326 Free PMC article.
-
The types and numbers of kinesins and dyneins transporting endocytic cargoes modulate their motility and response to tau.J Biol Chem. 2024 Jun;300(6):107323. doi: 10.1016/j.jbc.2024.107323. Epub 2024 Apr 25. J Biol Chem. 2024. PMID: 38677516 Free PMC article.
-
How Dynein Moves Along Microtubules.Trends Biochem Sci. 2016 Jan;41(1):94-105. doi: 10.1016/j.tibs.2015.11.004. Epub 2015 Dec 9. Trends Biochem Sci. 2016. PMID: 26678005 Free PMC article. Review.
-
Motor Skills: Recruitment of Kinesins, Myosins and Dynein during Assembly and Egress of Alphaherpesviruses.Viruses. 2021 Aug 17;13(8):1622. doi: 10.3390/v13081622. Viruses. 2021. PMID: 34452486 Free PMC article. Review.
Cited by
-
Endolysosomal vesicles at the center of B cell activation.J Cell Biol. 2024 Mar 4;223(3):e202307047. doi: 10.1083/jcb.202307047. Epub 2024 Feb 2. J Cell Biol. 2024. PMID: 38305771 Free PMC article. Review.
-
Key residues on microtubule responsible for activation of kinesin ATPase.EMBO J. 2010 Apr 7;29(7):1167-75. doi: 10.1038/emboj.2010.25. Epub 2010 Mar 11. EMBO J. 2010. PMID: 20224548 Free PMC article.
-
Phosphorylation-independent dual-site binding of the FHA domain of KIF13 mediates phosphoinositide transport via centaurin alpha1.Proc Natl Acad Sci U S A. 2010 Nov 23;107(47):20346-51. doi: 10.1073/pnas.1009008107. Epub 2010 Nov 5. Proc Natl Acad Sci U S A. 2010. PMID: 21057110 Free PMC article.
-
The auto-inhibitory domain and ATP-independent microtubule-binding region of Kinesin heavy chain are major functional domains for transport in the Drosophila germline.Development. 2014 Jan;141(1):176-86. doi: 10.1242/dev.097592. Epub 2013 Nov 20. Development. 2014. PMID: 24257625 Free PMC article.
-
These motors were made for walking.Protein Sci. 2020 Aug;29(8):1707-1723. doi: 10.1002/pro.3895. Epub 2020 Jun 26. Protein Sci. 2020. PMID: 32472639 Free PMC article. Review.
References
-
- Vale RD. The molecular motor toolbox for intracellular transport. Cell. 2003;112:467–480. - PubMed
-
- Vallee RB, Williams JC, Varma D, Barnhart LE. Dynein: an ancient motor protein involved in multiple modes of transport. J Neurobiol. 2004;58:189–200. - PubMed
-
- Howard J, Hudspeth AJ, Vale RD. Movement of microtubules by single kinesin molecules. Nature. 1989;342:154–158. - PubMed
-
- Block SM, Goldstein LS, Schnapp BJ. Bead movement by single kinesin molecules studied with optical tweezers. Nature. 1990;348:348–352. - PubMed
Publication types
MeSH terms
Substances
Grants and funding
LinkOut - more resources
Full Text Sources
Other Literature Sources