The novel lipid raft adaptor p18 controls endosome dynamics by anchoring the MEK-ERK pathway to late endosomes
- PMID: 19177150
- PMCID: PMC2657578
- DOI: 10.1038/emboj.2008.308
The novel lipid raft adaptor p18 controls endosome dynamics by anchoring the MEK-ERK pathway to late endosomes
Abstract
The regulation of endosome dynamics is crucial for fundamental cellular functions, such as nutrient intake/digestion, membrane protein cycling, cell migration and intracellular signalling. Here, we show that a novel lipid raft adaptor protein, p18, is involved in controlling endosome dynamics by anchoring the MEK1-ERK pathway to late endosomes. p18 is anchored to lipid rafts of late endosomes through its N-terminal unique region. p18(-/-) mice are embryonic lethal and have severe defects in endosome/lysosome organization and membrane protein transport in the visceral endoderm. p18(-/-) cells exhibit apparent defects in endosome dynamics through perinuclear compartment, such as aberrant distribution and/or processing of lysosomes and impaired cycling of Rab11-positive recycling endosomes. p18 specifically binds to the p14-MP1 complex, a scaffold for MEK1. Loss of p18 function excludes the p14-MP1 complex from late endosomes, resulting in a downregulation of the MEK-ERK activity. These results indicate that the lipid raft adaptor p18 is essential for anchoring the MEK-ERK pathway to late endosomes, and shed new light on a role of endosomal MEK-ERK pathway in controlling endosome dynamics.
Figures
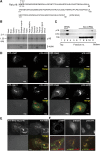
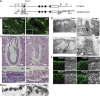
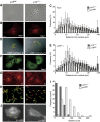
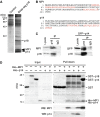
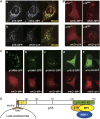
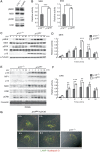
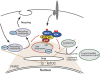
Similar articles
-
The late endosome/lysosome-anchored p18-mTORC1 pathway controls terminal maturation of lysosomes.Biochem Biophys Res Commun. 2012 Jan 27;417(4):1151-7. doi: 10.1016/j.bbrc.2011.12.082. Epub 2011 Dec 29. Biochem Biophys Res Commun. 2012. PMID: 22227194
-
Interactions between kinase scaffold MP1/p14 and its endosomal anchoring protein p18.Biochemistry. 2011 May 10;50(18):3696-705. doi: 10.1021/bi101972y. Epub 2011 Apr 15. Biochemistry. 2011. PMID: 21452851
-
p18/LAMTOR1: a late endosome/lysosome-specific anchor protein for the mTORC1/MAPK signaling pathway.Methods Enzymol. 2014;535:249-63. doi: 10.1016/B978-0-12-397925-4.00015-8. Methods Enzymol. 2014. PMID: 24377928
-
Caveosomes and endocytosis of lipid rafts.J Cell Sci. 2003 Dec 1;116(Pt 23):4707-14. doi: 10.1242/jcs.00840. J Cell Sci. 2003. PMID: 14600257 Review.
-
Molecular assemblies and membrane domains in multivesicular endosome dynamics.Exp Cell Res. 2009 May 15;315(9):1567-73. doi: 10.1016/j.yexcr.2008.12.006. Epub 2008 Dec 24. Exp Cell Res. 2009. PMID: 19133258 Review.
Cited by
-
LAMTOR1 ablation impedes cGAS degradation caused by chemotherapy and promotes antitumor immunity.Proc Natl Acad Sci U S A. 2024 Oct 8;121(41):e2320591121. doi: 10.1073/pnas.2320591121. Epub 2024 Oct 3. Proc Natl Acad Sci U S A. 2024. PMID: 39361643
-
Rag-Ragulator is the central organizer of the physical architecture of the mTORC1 nutrient-sensing pathway.Proc Natl Acad Sci U S A. 2024 Aug 27;121(35):e2322755121. doi: 10.1073/pnas.2322755121. Epub 2024 Aug 20. Proc Natl Acad Sci U S A. 2024. PMID: 39163330
-
TRAF4-Mediated LAMTOR1 Ubiquitination Promotes mTORC1 Activation and Inhibits the Inflammation-Induced Colorectal Cancer Progression.Adv Sci (Weinh). 2024 Mar;11(12):e2301164. doi: 10.1002/advs.202301164. Epub 2024 Jan 16. Adv Sci (Weinh). 2024. PMID: 38229144 Free PMC article.
-
Inhibition of lysosome-tethered Ragulator-Rag-3D complex restricts the replication of Enterovirus 71 and Coxsackie A16.J Cell Biol. 2023 Dec 4;222(12):e202303108. doi: 10.1083/jcb.202303108. Epub 2023 Oct 31. J Cell Biol. 2023. PMID: 37906052 Free PMC article.
-
The post-translational regulation of transcription factor EB (TFEB) in health and disease.EMBO Rep. 2023 Nov 6;24(11):e57574. doi: 10.15252/embr.202357574. Epub 2023 Sep 20. EMBO Rep. 2023. PMID: 37728021 Free PMC article. Review.
References
-
- Anderson RG, Jacobson K (2002) A role for lipid shells in targeting proteins to caveolae, rafts, and other lipid domains. Science 296: 1821–1825 - PubMed
-
- Balbis A, Parmar A, Wang Y, Baquiran G, Posner BI (2007) Compartmentalization of signaling-competent epidermal growth factor receptors in endosomes. Endocrinology 148: 2944–2954 - PubMed
-
- Bielinska M, Narita N, Wilson DB (1999) Distinct roles for visceral endoderm during embryonic mouse development. Int J Dev Biol 43: 183–205 - PubMed
-
- Bohn G, Allroth A, Brandes G, Thiel J, Glocker E, Schaffer AA, Rathinam C, Taub N, Teis D, Zeidler C, Dewey RA, Geffers R, Buer J, Huber LA, Welte K, Grimbacher B, Klein C (2007) A novel human primary immunodeficiency syndrome caused by deficiency of the endosomal adaptor protein p14. Nat Med 13: 38–45 - PubMed
Publication types
MeSH terms
Substances
LinkOut - more resources
Full Text Sources
Molecular Biology Databases
Research Materials
Miscellaneous