Conformational flexibility of metazoan fatty acid synthase enables catalysis
- PMID: 19151726
- PMCID: PMC2653270
- DOI: 10.1038/nsmb.1532
Conformational flexibility of metazoan fatty acid synthase enables catalysis
Abstract
The metazoan cytosolic fatty acid synthase (FAS) contains all of the enzymes required for de novo fatty acid biosynthesis covalently linked around two reaction chambers. Although the three-dimensional architecture of FAS has been mostly defined, it is unclear how reaction intermediates can transfer between distant catalytic domains. Using single-particle EM, we have identified a near continuum of conformations consistent with a remarkable flexibility of FAS. The distribution of conformations was influenced by the presence of substrates and altered by different catalytic mutations, suggesting a direct correlation between conformation and specific enzymatic activities. We interpreted three-dimensional reconstructions by docking high-resolution structures of individual domains, and they show that the substrate-loading and condensation domains dramatically swing and swivel to access substrates within either reaction chamber. Concomitant rearrangement of the beta-carbon-processing domains synchronizes acyl chain reduction in one chamber with acyl chain elongation in the other.
Figures
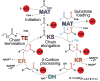
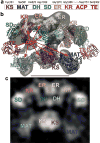
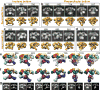
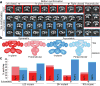
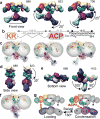
Similar articles
-
Single-particle electron microscopy of animal fatty acid synthase describing macromolecular rearrangements that enable catalysis.Methods Enzymol. 2010;483:179-202. doi: 10.1016/S0076-6879(10)83009-5. Methods Enzymol. 2010. PMID: 20888475
-
Regulating effect of β-ketoacyl synthase domain of fatty acid synthase on fatty acyl chain length in de novo fatty acid synthesis.Biochim Biophys Acta. 2016 Mar;1861(3):149-55. doi: 10.1016/j.bbalip.2015.12.002. Epub 2015 Dec 9. Biochim Biophys Acta. 2016. PMID: 26680361
-
Direct structural insight into the substrate-shuttling mechanism of yeast fatty acid synthase by electron cryomicroscopy.Proc Natl Acad Sci U S A. 2010 May 18;107(20):9164-9. doi: 10.1073/pnas.0913547107. Epub 2010 Mar 15. Proc Natl Acad Sci U S A. 2010. PMID: 20231485 Free PMC article.
-
Structural and functional organization of the animal fatty acid synthase.Prog Lipid Res. 2003 Jul;42(4):289-317. doi: 10.1016/s0163-7827(02)00067-x. Prog Lipid Res. 2003. PMID: 12689621 Review.
-
Structure and function of animal fatty acid synthase.Lipids. 2004 Nov;39(11):1045-53. doi: 10.1007/s11745-004-1329-9. Lipids. 2004. PMID: 15726818 Review.
Cited by
-
A conserved Mediator-CDK8 kinase module association regulates Mediator-RNA polymerase II interaction.Nat Struct Mol Biol. 2013 May;20(5):611-9. doi: 10.1038/nsmb.2549. Epub 2013 Apr 7. Nat Struct Mol Biol. 2013. PMID: 23563140 Free PMC article.
-
Direct structural analysis of a single acyl carrier protein domain in fatty acid synthase from the fungus Saccharomyces cerevisiae.Commun Biol. 2024 Jan 12;7(1):92. doi: 10.1038/s42003-024-05777-7. Commun Biol. 2024. PMID: 38216676 Free PMC article.
-
C-N bond formation by a polyketide synthase.Nat Commun. 2023 Mar 10;14(1):1319. doi: 10.1038/s41467-023-36989-w. Nat Commun. 2023. PMID: 36899013 Free PMC article.
-
Structural basis for the biosynthesis of lovastatin.Nat Commun. 2021 Feb 8;12(1):867. doi: 10.1038/s41467-021-21174-8. Nat Commun. 2021. PMID: 33558520 Free PMC article.
-
Structural interconversions modulate activity of Escherichia coli ribonucleotide reductase.Proc Natl Acad Sci U S A. 2011 Dec 27;108(52):21046-51. doi: 10.1073/pnas.1112715108. Epub 2011 Dec 12. Proc Natl Acad Sci U S A. 2011. PMID: 22160671 Free PMC article.
References
-
- Sul HS, Smith S. Fatty acid synthesis in eukaryotes. In: Vance DE, V JE, editors. Biochemistry of lipids, lipoproteins and membranes. Elsevier; 2008. pp. 155–190.
-
- Loftus TM, et al. Reduced food intake and body weight in mice treated with fatty acid synthase inhibitors. Science. 2000;288:2379–81. - PubMed
-
- Jenni S, et al. Structure of fungal fatty acid synthase and implications for iterative substrate shuttling. Science. 2007;316:254–61. - PubMed
-
- Lomakin IB, Xiong Y, Steitz TA. The crystal structure of yeast fatty acid synthase, a cellular machine with eight active sites working together. Cell. 2007;129:319–32. - PubMed
Publication types
MeSH terms
Substances
Grants and funding
LinkOut - more resources
Full Text Sources
Other Literature Sources
Molecular Biology Databases
Research Materials
Miscellaneous