An upstream open reading frame regulates translation of GADD34 during cellular stresses that induce eIF2alpha phosphorylation
- PMID: 19131336
- PMCID: PMC2652341
- DOI: 10.1074/jbc.M806735200
An upstream open reading frame regulates translation of GADD34 during cellular stresses that induce eIF2alpha phosphorylation
Abstract
Cellular stress such as endoplasmic reticulum stress, hypoxia, and viral infection activates an integrated stress response, which includes the phosphorylation of the eukaryotic initiation factor 2alpha (eIF2alpha) to inhibit overall protein synthesis. Paradoxically, this leads to translation of a subset of mRNAs, like transcription factor ATF4, which in turn induces transcription of downstream stress-induced genes such as growth arrest DNA-inducible gene 34 (GADD34). GADD34 interacts with protein phosphatase 1 to dephosphorylate eIF2alpha, resulting in a negative feedback loop to recover protein synthesis and allow translation of stress-induced transcripts. Here, we show that GADD34 is not only transcriptionally induced but also translationally regulated to ensure maximal expression during eIF2alpha phosphorylation. GADD34 mRNAs are preferentially associated with polysomes during eIF2alpha phosphorylation, which is mediated by its 5'-untranslated region (5'UTR). The human GADD34 5'UTR contains two non-overlapping upstream open reading frames (uORFs), whereas the mouse version contains two overlapping and out of frame uORFs. Using 5'UTR GADD34 reporter constructs, we show that the downstream uORF mediates repression of basal translation and directs translation during eIF2alpha phosphorylation. Furthermore, we show that the upstream uORF is poorly translated and that a proportion of scanning ribosomes bypasses the upstream uORF to recognize the downstream uORF. These findings suggest that GADD34 translation is regulated by a unique 5'UTR uORF mechanism to ensure proper GADD34 expression during eIF2alpha phosphorylation. This mechanism may serve as a model for understanding how other 5'UTR uORF-containing mRNAs are regulated during cellular stress.
Figures
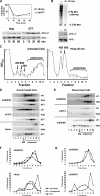
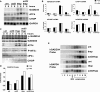
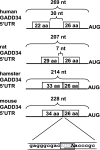
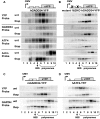
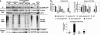
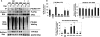
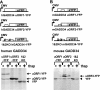
Similar articles
-
Ribosome Reinitiation Directs Gene-specific Translation and Regulates the Integrated Stress Response.J Biol Chem. 2015 Nov 20;290(47):28257-28271. doi: 10.1074/jbc.M115.693184. Epub 2015 Oct 7. J Biol Chem. 2015. PMID: 26446796 Free PMC article.
-
The 5'-untranslated region of multidrug resistance associated protein 2 (MRP2; ABCC2) regulates downstream open reading frame expression through translational regulation.Mol Pharmacol. 2010 Feb;77(2):237-46. doi: 10.1124/mol.109.058982. Epub 2009 Nov 4. Mol Pharmacol. 2010. PMID: 19890061 Free PMC article.
-
Regulation of internal ribosome entry site-mediated translation by eukaryotic initiation factor-2alpha phosphorylation and translation of a small upstream open reading frame.J Biol Chem. 2002 Jan 18;277(3):2050-8. doi: 10.1074/jbc.M109199200. Epub 2001 Oct 29. J Biol Chem. 2002. PMID: 11684693
-
Some like it translated: small ORFs in the 5'UTR.Exp Cell Res. 2020 Nov 1;396(1):112229. doi: 10.1016/j.yexcr.2020.112229. Epub 2020 Aug 17. Exp Cell Res. 2020. PMID: 32818479 Review.
-
Upstream Open Reading Frames Differentially Regulate Gene-specific Translation in the Integrated Stress Response.J Biol Chem. 2016 Aug 12;291(33):16927-35. doi: 10.1074/jbc.R116.733899. Epub 2016 Jun 29. J Biol Chem. 2016. PMID: 27358398 Free PMC article. Review.
Cited by
-
A perspective on mammalian upstream open reading frame function.Int J Biochem Cell Biol. 2013 Aug;45(8):1690-700. doi: 10.1016/j.biocel.2013.04.020. Epub 2013 Apr 25. Int J Biochem Cell Biol. 2013. PMID: 23624144 Free PMC article. Review.
-
GADD34 is a modulator of autophagy during starvation.Sci Adv. 2020 Sep 25;6(39):eabb0205. doi: 10.1126/sciadv.abb0205. Print 2020 Sep. Sci Adv. 2020. PMID: 32978159 Free PMC article.
-
The transcription factor network associated with the amino acid response in mammalian cells.Adv Nutr. 2012 May 1;3(3):295-306. doi: 10.3945/an.112.001891. Adv Nutr. 2012. PMID: 22585903 Free PMC article. Review.
-
Specific activation of the integrated stress response uncovers regulation of central carbon metabolism and lipid droplet biogenesis.Nat Commun. 2024 Sep 27;15(1):8301. doi: 10.1038/s41467-024-52538-5. Nat Commun. 2024. PMID: 39333061 Free PMC article.
-
A data-entrained computational model for testing the regulatory logic of the vertebrate unfolded protein response.Mol Biol Cell. 2018 Jun 15;29(12):1502-1517. doi: 10.1091/mbc.E17-09-0565. Epub 2018 Apr 18. Mol Biol Cell. 2018. PMID: 29668363 Free PMC article.
References
-
- Ron, D., and Harding, H. P. (2007) in Translational Control in Biology and Medicine (Mathews, M. B., Sonenberg, N., and Hershey, J., eds) pp. 345–368, Cold Spring Harbor Laboratory Press, Cold Spring Harbor, NY
-
- Dever, T. E., Dar, A. C., and Sicheri, F. (2007) in Translational Control in Biology and Medicine (Mathews, M. B., Sonenberg, N., and Hershey, J., eds) pp. 319–344, Cold Spring Harbor Laboratory Press, Cold Spring Harbor, NY
Publication types
MeSH terms
Substances
LinkOut - more resources
Full Text Sources
Other Literature Sources
Molecular Biology Databases
Miscellaneous