The microtubule-binding protein CLIP-170 coordinates mDia1 and actin reorganization during CR3-mediated phagocytosis
- PMID: 19114595
- PMCID: PMC2606960
- DOI: 10.1083/jcb.200807023
The microtubule-binding protein CLIP-170 coordinates mDia1 and actin reorganization during CR3-mediated phagocytosis
Abstract
Microtubule dynamics are modulated by regulatory proteins that bind to their plus ends (+TIPs [plus end tracking proteins]), such as cytoplasmic linker protein 170 (CLIP-170) or end-binding protein 1 (EB1). We investigated the role of +TIPs during phagocytosis in macrophages. Using RNA interference and dominant-negative approaches, we show that CLIP-170 is specifically required for efficient phagocytosis triggered by alphaMbeta2 integrin/complement receptor activation. This property is not observed for EB1 and EB3. Accordingly, whereas CLIP-170 is dynamically enriched at the site of phagocytosis, EB1 is not. Furthermore, we observe that CLIP-170 controls the recruitment of the formin mDia1, an actin-nucleating protein, at the onset of phagocytosis and thereby controls actin polymerization events that are essential for phagocytosis. CLIP-170 directly interacts with the formin homology 2 domain of mDia1. The interaction between CLIP-170 and mDia1 is negatively regulated during alphaMbeta2-mediated phagocytosis. Our results unravel a new microtubule/actin cooperation that involves CLIP-170 and mDia1 and that functions downstream of alphaMbeta2 integrins.
Figures
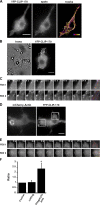
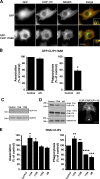
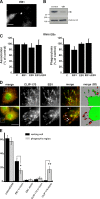
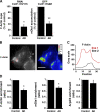
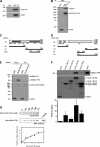
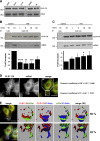
Similar articles
-
Differential interactions of the formins INF2, mDia1, and mDia2 with microtubules.Mol Biol Cell. 2011 Dec;22(23):4575-87. doi: 10.1091/mbc.E11-07-0616. Epub 2011 Oct 12. Mol Biol Cell. 2011. PMID: 21998204 Free PMC article.
-
A role for mammalian diaphanous-related formins in complement receptor (CR3)-mediated phagocytosis in macrophages.Curr Biol. 2005 Nov 22;15(22):2007-12. doi: 10.1016/j.cub.2005.09.051. Curr Biol. 2005. PMID: 16303559
-
Essential and nonredundant roles for Diaphanous formins in cortical microtubule capture and directed cell migration.Mol Biol Cell. 2014 Mar;25(5):658-68. doi: 10.1091/mbc.E13-08-0482. Epub 2014 Jan 8. Mol Biol Cell. 2014. PMID: 24403606 Free PMC article.
-
Actin-capping protein promotes microtubule stability by antagonizing the actin activity of mDia1.Mol Biol Cell. 2012 Oct;23(20):4032-40. doi: 10.1091/mbc.E12-05-0338. Epub 2012 Aug 23. Mol Biol Cell. 2012. PMID: 22918941 Free PMC article.
-
FORMIN a link between kinetochores and microtubule ends.Trends Cell Biol. 2011 Nov;21(11):625-9. doi: 10.1016/j.tcb.2011.08.005. Epub 2011 Sep 13. Trends Cell Biol. 2011. PMID: 21920754 Free PMC article. Review.
Cited by
-
mDia1-3 in mammalian filopodia.Commun Integr Biol. 2012 Jul 1;5(4):340-4. doi: 10.4161/cib.20214. Commun Integr Biol. 2012. PMID: 23060957 Free PMC article.
-
Unleashing formins to remodel the actin and microtubule cytoskeletons.Nat Rev Mol Cell Biol. 2010 Jan;11(1):62-74. doi: 10.1038/nrm2816. Epub 2009 Dec 9. Nat Rev Mol Cell Biol. 2010. PMID: 19997130 Review.
-
Microtubules regulate brush border formation.J Cell Physiol. 2018 Feb;233(2):1468-1480. doi: 10.1002/jcp.26033. Epub 2017 Aug 4. J Cell Physiol. 2018. PMID: 28548701 Free PMC article.
-
Phagocytic Integrins: Activation and Signaling.Front Immunol. 2020 Apr 30;11:738. doi: 10.3389/fimmu.2020.00738. eCollection 2020. Front Immunol. 2020. PMID: 32425937 Free PMC article. Review.
-
Formins and microtubules.Biochim Biophys Acta. 2010 Feb;1803(2):164-73. doi: 10.1016/j.bbamcr.2009.07.006. Epub 2009 Jul 23. Biochim Biophys Acta. 2010. PMID: 19631698 Free PMC article. Review.
References
-
- Aderem, A., and D.M. Underhill. 1999. Mechanisms of phagocytosis in macrophages. Annu. Rev. Immunol. 17:593–623. - PubMed
-
- Akhmanova, A., and M.O. Steinmetz. 2008. Tracking the ends: a dynamic protein network controls the fate of microtubule tips. Nat. Rev. Mol. Cell Biol. 9:309–322. - PubMed
-
- Araki, N., T. Hatae, A. Furukawa, and J.A. Swanson. 2003. Phosphoinositide-3-kinase-independent contractile activities associated with Fcgamma-receptor-mediated phagocytosis and macropinocytosis in macrophages. J. Cell Sci. 116:247–257. - PubMed
Publication types
MeSH terms
Substances
LinkOut - more resources
Full Text Sources
Molecular Biology Databases
Research Materials