Reactive oxygen species regulate F-actin dynamics in neuronal growth cones and neurite outgrowth
- PMID: 19054285
- PMCID: PMC2995541
- DOI: 10.1111/j.1471-4159.2008.05787.x
Reactive oxygen species regulate F-actin dynamics in neuronal growth cones and neurite outgrowth
Abstract
Reactive oxygen species are well known for their damaging effects due to oxidation of lipids, proteins and DNA that ultimately result in cell death. Accumulating evidence indicates that reactive oxygen species also have important signaling functions in cell proliferation, differentiation, cell motility and apoptosis. Here, we tested the hypothesis whether reactive oxygen species play a physiological role in regulating F-actin structure and dynamics in neuronal growth cones. Lowering cytoplasmic levels of reactive oxygen species with a free radical scavenger, N-tert-butyl-alpha-phenylnitrone, or by inhibiting specific sources of reactive oxygen species, such as NADPH oxidases or lipoxygenases, reduced the F-actin content in the peripheral domain of growth cones. Fluorescent speckle microscopy revealed that these treatments caused actin assembly inhibition, reduced retrograde actin flow and increased contractility of actin structures in the transition zone referred to as arcs, possibly by activating the Rho pathway. Reduced levels of reactive oxygen species ultimately resulted in disassembly of the actin cytoskeleton. When neurons were cultured overnight in conditions of reduced free radicals, growth cone formation and neurite outgrowth were severely impaired. Therefore, we conclude that physiological levels of reactive oxygen species are critical for maintaining a dynamic F-actin cytoskeleton and controlling neurite outgrowth.
Figures
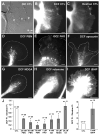
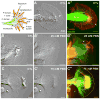
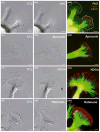
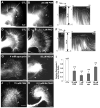
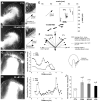
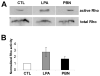
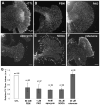
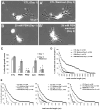
Similar articles
-
Bidirectional interactions between NOX2-type NADPH oxidase and the F-actin cytoskeleton in neuronal growth cones.J Neurochem. 2014 Aug;130(4):526-40. doi: 10.1111/jnc.12734. Epub 2014 Apr 25. J Neurochem. 2014. PMID: 24702317 Free PMC article.
-
Neuronal NADPH oxidase is required for neurite regeneration of Aplysia bag cell neurons.J Neurochem. 2023 Nov;167(4):505-519. doi: 10.1111/jnc.15977. Epub 2023 Oct 11. J Neurochem. 2023. PMID: 37818836 Free PMC article.
-
Rac1-dependent actin filament organization in growth cones is necessary for beta1-integrin-mediated advance but not for growth on poly-D-lysine.J Neurobiol. 1998 Dec;37(4):524-40. doi: 10.1002/(sici)1097-4695(199812)37:4<524::aid-neu3>3.0.co;2-h. J Neurobiol. 1998. PMID: 9858256
-
Regulation of actin filament assembly and disassembly in growth cone motility and axon guidance.Brain Res Bull. 2023 Jan;192:21-35. doi: 10.1016/j.brainresbull.2022.10.019. Epub 2022 Nov 3. Brain Res Bull. 2023. PMID: 36336143 Review.
-
Regulating actin dynamics in neuronal growth cones by ADF/cofilin and rho family GTPases.J Neurobiol. 2000 Aug;44(2):126-44. J Neurobiol. 2000. PMID: 10934317 Review.
Cited by
-
Nystatin Regulates Axonal Extension and Regeneration by Modifying the Levels of Nitric Oxide.Front Mol Neurosci. 2020 Apr 3;13:56. doi: 10.3389/fnmol.2020.00056. eCollection 2020. Front Mol Neurosci. 2020. PMID: 32317932 Free PMC article.
-
Bidirectional interactions between NOX2-type NADPH oxidase and the F-actin cytoskeleton in neuronal growth cones.J Neurochem. 2014 Aug;130(4):526-40. doi: 10.1111/jnc.12734. Epub 2014 Apr 25. J Neurochem. 2014. PMID: 24702317 Free PMC article.
-
Invasive cells in animals and plants: searching for LECA machineries in later eukaryotic life.Biol Direct. 2013 Apr 4;8:8. doi: 10.1186/1745-6150-8-8. Biol Direct. 2013. PMID: 23557484 Free PMC article. Review.
-
Mechanical cues protect against silica nanoparticle exposure in SH-SY5Y neuroblastoma.Toxicol In Vitro. 2021 Feb;70:105031. doi: 10.1016/j.tiv.2020.105031. Epub 2020 Oct 17. Toxicol In Vitro. 2021. PMID: 33075489 Free PMC article.
-
Polyunsaturated Fatty Acid Composition of Cerebrospinal Fluid Fractions Shows Their Contribution to Cognitive Resilience of a Pre-symptomatic Alzheimer's Disease Cohort.Front Physiol. 2020 Feb 14;11:83. doi: 10.3389/fphys.2020.00083. eCollection 2020. Front Physiol. 2020. PMID: 32116789 Free PMC article.
References
-
- Abo A, Pick E, Hall A, Totty N, Teahan CG, Segal AW. Activation of the NADPH oxidase involves the small GTP-binding protein p21rac1. Nature. 1991;353:668–670. - PubMed
-
- Alexandrova AY, Kopnin PB, Vasiliev JM, Kopnin BP. ROS up-regulation mediates Ras-induced changes of cell morphology and motility. Exp Cell Res. 2006;312:2066–2073. - PubMed
-
- Barrientos A, Moraes CT. Titrating the effects of mitochondrial complex I impairment in the cell physiology. J Biol Chem. 1999;274:16188–16197. - PubMed
-
- Bedard K, Krause KH. The NOX family of ROS-generating NADPH oxidases: physiology and pathophysiology. Physiol Rev. 2007;87:245–313. - PubMed
Publication types
MeSH terms
Substances
Grants and funding
LinkOut - more resources
Full Text Sources