Chimeric human parainfluenza virus bearing the Ebola virus glycoprotein as the sole surface protein is immunogenic and highly protective against Ebola virus challenge
- PMID: 19010509
- PMCID: PMC2649782
- DOI: 10.1016/j.virol.2008.09.030
Chimeric human parainfluenza virus bearing the Ebola virus glycoprotein as the sole surface protein is immunogenic and highly protective against Ebola virus challenge
Abstract
We generated a new live-attenuated vaccine against Ebola virus (EBOV) based on a chimeric virus HPIV3/DeltaF-HN/EboGP that contains the EBOV glycoprotein (GP) as the sole transmembrane envelope protein combined with the internal proteins of human parainfluenza virus type 3 (HPIV3). Electron microscopy analysis of the virus particles showed that they have an envelope and surface spikes resembling those of EBOV and a particle size and shape resembling those of HPIV3. When HPIV3/DeltaF-HN/EboGP was inoculated via apical surface of an in vitro model of human ciliated airway epithelium, the virus was released from the apical surface; when applied to basolateral surface, the virus infected basolateral cells but did not spread through the tissue. Following intranasal (IN) inoculation of guinea pigs, scattered infected cells were detected in the lungs by immunohistochemistry, but infectious HPIV3/DeltaF-HN/EboGP could not be recovered from the lungs, blood, or other tissues. Despite the attenuation, the virus was highly immunogenic, and a single IN dose completely protected the animals against a highly lethal intraperitoneal challenge of guinea pig-adapted EBOV.
Figures
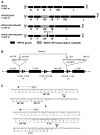
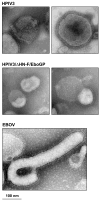
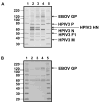
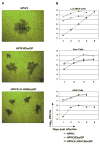
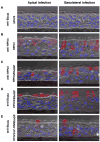
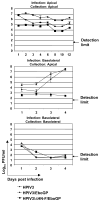
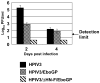
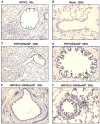
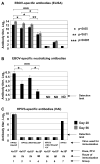
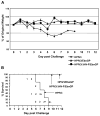
Similar articles
-
A single intranasal inoculation with a paramyxovirus-vectored vaccine protects guinea pigs against a lethal-dose Ebola virus challenge.J Virol. 2006 Mar;80(5):2267-79. doi: 10.1128/JVI.80.5.2267-2279.2006. J Virol. 2006. PMID: 16474134 Free PMC article.
-
Attenuated Human Parainfluenza Virus Type 1 Expressing Ebola Virus Glycoprotein GP Administered Intranasally Is Immunogenic in African Green Monkeys.J Virol. 2017 Apr 28;91(10):e02469-16. doi: 10.1128/JVI.02469-16. Print 2017 May 15. J Virol. 2017. PMID: 28250127 Free PMC article.
-
Mucosal parainfluenza virus-vectored vaccine against Ebola virus replicates in the respiratory tract of vector-immune monkeys and is immunogenic.Virology. 2010 Apr 10;399(2):290-8. doi: 10.1016/j.virol.2010.01.015. Epub 2010 Feb 2. Virology. 2010. PMID: 20129638 Free PMC article.
-
The vesicular stomatitis virus-based Ebola virus vaccine: From concept to clinical trials.Hum Vaccin Immunother. 2018;14(9):2107-2113. doi: 10.1080/21645515.2018.1473698. Epub 2018 Jun 18. Hum Vaccin Immunother. 2018. PMID: 29757706 Free PMC article. Review.
-
Correlates of vaccine-induced protective immunity against Ebola virus disease.Semin Immunol. 2018 Oct;39:65-72. doi: 10.1016/j.smim.2018.07.003. Epub 2018 Jul 21. Semin Immunol. 2018. PMID: 30041831 Review.
Cited by
-
Emerging targets and novel approaches to Ebola virus prophylaxis and treatment.BioDrugs. 2013 Dec;27(6):565-83. doi: 10.1007/s40259-013-0046-1. BioDrugs. 2013. PMID: 23813435 Free PMC article. Review.
-
Structural and Functional Aspects of Ebola Virus Proteins.Pathogens. 2021 Oct 15;10(10):1330. doi: 10.3390/pathogens10101330. Pathogens. 2021. PMID: 34684279 Free PMC article. Review.
-
The lack of maturation of Ebola virus-infected dendritic cells results from the cooperative effect of at least two viral domains.J Virol. 2013 Jul;87(13):7471-85. doi: 10.1128/JVI.03316-12. Epub 2013 Apr 24. J Virol. 2013. PMID: 23616668 Free PMC article.
-
Ebola virus disease candidate vaccines under evaluation in clinical trials.Expert Rev Vaccines. 2016 Sep;15(9):1101-12. doi: 10.1080/14760584.2016.1187566. Epub 2016 May 27. Expert Rev Vaccines. 2016. PMID: 27160784 Free PMC article. Review.
-
Potential vaccines and post-exposure treatments for filovirus infections.Viruses. 2012 Sep;4(9):1619-50. doi: 10.3390/v4091619. Epub 2012 Sep 21. Viruses. 2012. PMID: 23170176 Free PMC article. Review.
References
-
- Barouch DH, Pau MG, Custers JH, Koudstaal W, Kostense S, Havenga MJ, Truitt DM, Sumida SM, Kishko MG, Arthur JC, Korioth-Schmitz B, Newberg MH, Gorgone DA, Lifton MA, Panicali DL, Nabel GJ, Letvin NL, Goudsmit J. Immunogenicity of recombinant adenovirus serotype 35 vaccine in the presence of pre-existing anti-Ad5 immunity. J Immunol. 2004;172(10):6290–7. - PubMed
-
- Casimiro DR, Chen L, Fu TM, Evans RK, Caulfield MJ, Davies ME, Tang A, Chen M, Huang L, Harris V, Freed DC, Wilson KA, Dubey S, Zhu DM, Nawrocki D, Mach H, Troutman R, Isopi L, Williams D, Hurni W, Xu Z, Smith JG, Wang S, Liu X, Guan L, Long R, Trigona W, Heidecker GJ, Perry HC, Persaud N, Toner TJ, Su Q, Liang X, Youil R, Chastain M, Bett AJ, Volkin DB, Emini EA, Shiver JW. Comparative immunogenicity in rhesus monkeys of DNA plasmid, recombinant vaccinia virus, and replication-defective adenovirus vectors expressing a human immunodeficiency virus type 1 gag gene. J Virol. 2003;77(11):6305–13. - PMC - PubMed
Publication types
MeSH terms
Substances
Grants and funding
LinkOut - more resources
Full Text Sources
Other Literature Sources
Medical