Structural and functional studies of the promoter element for dengue virus RNA replication
- PMID: 19004935
- PMCID: PMC2612346
- DOI: 10.1128/JVI.01647-08
Structural and functional studies of the promoter element for dengue virus RNA replication
Abstract
The 5' untranslated region (5'UTR) of the dengue virus (DENV) genome contains two defined elements essential for viral replication. At the 5' end, a large stem-loop (SLA) structure functions as the promoter for viral polymerase activity. Next to the SLA, there is a short stem-loop that contains a cyclization sequence known as the 5' upstream AUG region (5'UAR). Here, we analyzed the secondary structure of the SLA in solution and the structural requirements of this element for viral replication. Using infectious DENV clones, viral replicons, and in vitro polymerase assays, we defined two helical regions, a side stem-loop, a top loop, and a U bulge within SLA as crucial elements for viral replication. The determinants for SLA-polymerase recognition were found to be common in different DENV serotypes. In addition, structural elements within the SLA required for DENV RNA replication were also conserved among different mosquito- and tick-borne flavivirus genomes, suggesting possible common strategies for polymerase-promoter recognition in flaviviruses. Furthermore, a conserved oligo(U) track present downstream of the SLA was found to modulate RNA synthesis in transfected cells. In vitro polymerase assays indicated that a sequence of at least 10 residues following the SLA, upstream of the 5'UAR, was necessary for efficient RNA synthesis using the viral 3'UTR as template.
Figures
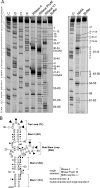
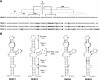
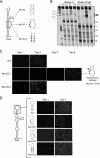
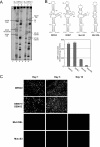
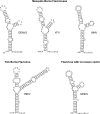
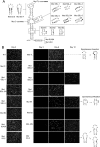
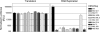
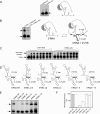
Similar articles
-
Interplay of RNA elements in the dengue virus 5' and 3' ends required for viral RNA replication.J Virol. 2010 Jun;84(12):6103-18. doi: 10.1128/JVI.02042-09. Epub 2010 Mar 31. J Virol. 2010. PMID: 20357095 Free PMC article.
-
Structure of the dengue virus RNA promoter.RNA. 2022 Sep;28(9):1210-1223. doi: 10.1261/rna.079197.122. Epub 2022 Jun 24. RNA. 2022. PMID: 35750488 Free PMC article.
-
Interactions between the Dengue Virus Polymerase NS5 and Stem-Loop A.J Virol. 2017 May 12;91(11):e00047-17. doi: 10.1128/JVI.00047-17. Print 2017 Jun 1. J Virol. 2017. PMID: 28356528 Free PMC article.
-
Functional RNA elements in the dengue virus genome.Viruses. 2011 Sep;3(9):1739-56. doi: 10.3390/v3091739. Epub 2011 Sep 15. Viruses. 2011. PMID: 21994804 Free PMC article. Review.
-
The Role of the Stem-Loop A RNA Promoter in Flavivirus Replication.Viruses. 2021 Jun 9;13(6):1107. doi: 10.3390/v13061107. Viruses. 2021. PMID: 34207869 Free PMC article. Review.
Cited by
-
Replacement of the heterologous 5' untranslated region allows preservation of the fully functional activities of type 2 porcine reproductive and respiratory syndrome virus.Virology. 2013 Apr 25;439(1):1-12. doi: 10.1016/j.virol.2012.12.013. Epub 2013 Mar 1. Virology. 2013. PMID: 23453581 Free PMC article.
-
A 3'-end structure in RNA2 of a crinivirus is essential for viral RNA synthesis and contributes to replication-associated translation activity.Sci Rep. 2016 Oct 3;6:34482. doi: 10.1038/srep34482. Sci Rep. 2016. PMID: 27694962 Free PMC article.
-
Molecular characterization and phylogenetic analysis of a dengue virus serotype 3 isolated from a Chinese traveler returned from Laos.Virol J. 2018 Jul 24;15(1):113. doi: 10.1186/s12985-018-1016-5. Virol J. 2018. PMID: 30041666 Free PMC article.
-
Endless Forms: Within-Host Variation in the Structure of the West Nile Virus RNA Genome during Serial Passage in Bird Hosts.mSphere. 2019 Jun 26;4(3):e00291-19. doi: 10.1128/mSphere.00291-19. mSphere. 2019. PMID: 31243074 Free PMC article.
-
Sequence duplication in 3' UTR modulates virus replication and virulence of Japanese encephalitis virus.Emerg Microbes Infect. 2022 Dec;11(1):123-135. doi: 10.1080/22221751.2021.2016354. Emerg Microbes Infect. 2022. PMID: 34877923 Free PMC article.
References
-
- Alvarez, D. E., A. L. De Lella Ezcurra, S. Fucito, and A. V. Gamarnik. 2005. Role of RNA structures present at the 3′UTR of dengue virus on translation, RNA synthesis, and viral replication. Virology 339200-212. - PubMed
-
- Alvarez, D. E., C. V. Filomatori, and A. V. Gamarnik. 2008. Functional analysis of dengue virus cyclization sequences located at the 5′ and 3′UTRs. Virology 375223-235. - PubMed
-
- Blaney, J. E., Jr., A. P. Durbin, B. R. Murphy, and S. S. Whitehead. 2006. Development of a live attenuated dengue virus vaccine using reverse genetics. Viral Immunol. 1910-32. - PubMed
-
- Blaney, J. E., Jr., N. S. Sathe, L. Goddard, C. T. Hanson, T. A. Romero, K. A. Hanley, B. R. Murphy, and S. S. Whitehead. 2008. Dengue virus type 3 vaccine candidates generated by introduction of deletions in the 3′ untranslated region (3′-UTR) or by exchange of the DENV-3 3′-UTR with that of DENV-4. Vaccine 26817-828. - PMC - PubMed
Publication types
MeSH terms
Substances
LinkOut - more resources
Full Text Sources