Signal regulatory proteins (SIRPS) are secreted presynaptic organizing molecules
- PMID: 18819922
- PMCID: PMC2662226
- DOI: 10.1074/jbc.M805729200
Signal regulatory proteins (SIRPS) are secreted presynaptic organizing molecules
Abstract
Formation of chemical synapses requires exchange of organizing signals between the synaptic partners. Using synaptic vesicle aggregation in cultured neurons as a marker of presynaptic differentiation, we purified candidate presynaptic organizers from mouse brain. A major bioactive species was the extracellular domain of signal regulatory protein alpha (SIRP-alpha), a transmembrane immunoglobulin superfamily member concentrated at synapses. The extracellular domain of SIRP-alpha is cleaved and shed in a developmentally regulated manner. The presynaptic organizing activity of SIRP-alpha is mediated in part by CD47. SIRP-alpha homologues, SIRP-beta and -gamma also have synaptic vesicle clustering activity. The effects of SIRP-alpha are distinct from those of another presynaptic organizer, FGF22: the two proteins induced vesicle clusters of different sizes, differed in their ability to promote neurite branching, and acted through different receptors and signaling pathways. SIRP family proteins may act together with other organizing molecules to pattern synapses.
Figures
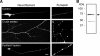
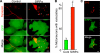
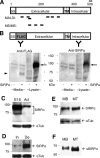
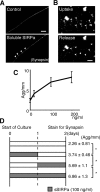
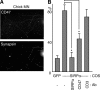
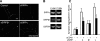
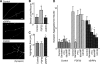
Similar articles
-
SIRP-alpha-CD47 system functions as an intercellular signal in the renal glomerulus.Am J Physiol Renal Physiol. 2010 Sep;299(3):F517-27. doi: 10.1152/ajprenal.00571.2009. Epub 2010 Jun 16. Am J Physiol Renal Physiol. 2010. PMID: 20554646
-
FGF22 and its close relatives are presynaptic organizing molecules in the mammalian brain.Cell. 2004 Jul 23;118(2):257-70. doi: 10.1016/j.cell.2004.06.025. Cell. 2004. PMID: 15260994
-
Novel structural determinants on SIRP alpha that mediate binding to CD47.J Immunol. 2007 Dec 1;179(11):7741-50. doi: 10.4049/jimmunol.179.11.7741. J Immunol. 2007. PMID: 18025220
-
Signal regulation by family conspiracy.Cell Mol Life Sci. 2001 Jan;58(1):117-24. doi: 10.1007/PL00000771. Cell Mol Life Sci. 2001. PMID: 11229810 Free PMC article. Review.
-
CD47 in the immune response: role of thrombospondin and SIRP-alpha reverse signaling.Curr Drug Targets. 2008 Oct;9(10):842-50. doi: 10.2174/138945008785909310. Curr Drug Targets. 2008. PMID: 18855618 Review.
Cited by
-
Distinct roles of muscle and motoneuron LRP4 in neuromuscular junction formation.Neuron. 2012 Jul 12;75(1):94-107. doi: 10.1016/j.neuron.2012.04.033. Neuron. 2012. PMID: 22794264 Free PMC article.
-
MicroRNA-206 delays ALS progression and promotes regeneration of neuromuscular synapses in mice.Science. 2009 Dec 11;326(5959):1549-54. doi: 10.1126/science.1181046. Science. 2009. PMID: 20007902 Free PMC article.
-
Leishmania Infection-Induced Proteolytic Processing of SIRPα in Macrophages.Pathogens. 2023 Apr 13;12(4):593. doi: 10.3390/pathogens12040593. Pathogens. 2023. PMID: 37111479 Free PMC article.
-
Lrp4 is a retrograde signal for presynaptic differentiation at neuromuscular synapses.Nature. 2012 Sep 20;489(7416):438-42. doi: 10.1038/nature11348. Epub 2012 Aug 1. Nature. 2012. PMID: 22854782 Free PMC article.
-
Signal regulatory protein alpha (SIRPalpha)/CD47 interaction and function.Curr Opin Immunol. 2009 Feb;21(1):47-52. doi: 10.1016/j.coi.2009.01.008. Epub 2009 Feb 14. Curr Opin Immunol. 2009. PMID: 19223164 Free PMC article. Review.
References
-
- Sanes, J. R., and Lichtman, J. W. (1999) Annu. Rev. Neurosci. 22 389-442 - PubMed
-
- Waites, C. L., Craig, A. M., and Garner, C. C. (2005) Annu. Rev. Neurosci. 28 251-274 - PubMed
-
- Fox, M. A., and Umemori, H. (2006) J. Neurochem. 97 1215-1231 - PubMed
-
- Scheiffele, P. (2003) Annu. Rev. Neurosci. 26 485-508 - PubMed
-
- Yamagata, M., Sanes, J. R., and Weiner, J. A. (2003) Curr. Opin. Cell Biol. 15 621-632 - PubMed
MeSH terms
Substances
LinkOut - more resources
Full Text Sources
Other Literature Sources
Molecular Biology Databases
Research Materials