Plasmodium falciparum Sir2 is an NAD+-dependent deacetylase and an acetyllysine-dependent and acetyllysine-independent NAD+ glycohydrolase
- PMID: 18729382
- PMCID: PMC2732577
- DOI: 10.1021/bi800767t
Plasmodium falciparum Sir2 is an NAD+-dependent deacetylase and an acetyllysine-dependent and acetyllysine-independent NAD+ glycohydrolase
Abstract
Sirtuins are NAD (+)-dependent enzymes that deacetylate a variety of cellular proteins and in some cases catalyze protein ADP-ribosyl transfer. The catalytic mechanism of deacetylation is proposed to involve an ADPR-peptidylimidate, whereas the mechanism of ADP-ribosyl transfer to proteins is undetermined. Herein we characterize a Plasmodium falciparum sirtuin that catalyzes deacetylation of histone peptide sequences. Interestingly, the enzyme can also hydrolyze NAD (+). Two mechanisms of hydrolysis were identified and characterized. One is independent of acetyllysine substrate and produces alpha-stereochemistry as established by reaction of methanol which forms alpha-1- O-methyl-ADPR. This reaction is insensitive to nicotinamide inhibition. The second solvolytic mechanism is dependent on acetylated peptide and is proposed to involve the imidate to generate beta-stereochemistry. Stereochemistry was established by isolation of beta-1- O-methyl-ADPR when methanol was added as a cosolvent. This solvolytic reaction was inhibited by nicotinamide, suggesting that nicotinamide and solvent compete for the imidate. These findings establish new reactions of wildtype sirtuins and suggest possible mechanisms for ADP-ribosylation to proteins. These findings also illustrate the potential utility of nicotinamide as a probe for mechanisms of sirtuin-catalyzed ADP-ribosyl transfer.
Figures
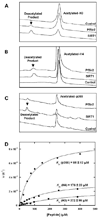
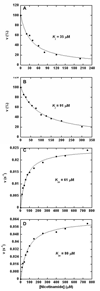
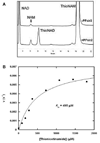
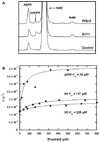
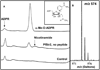
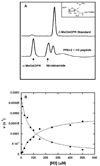
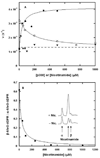
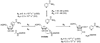
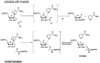
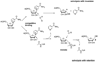
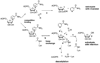
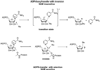
Similar articles
-
Acetylation-dependent ADP-ribosylation by Trypanosoma brucei Sir2.J Biol Chem. 2008 Feb 29;283(9):5317-26. doi: 10.1074/jbc.M707613200. Epub 2007 Dec 27. J Biol Chem. 2008. PMID: 18165239
-
Plasmodium falciparum Sir2: an unusual sirtuin with dual histone deacetylase and ADP-ribosyltransferase activity.Eukaryot Cell. 2007 Nov;6(11):2081-91. doi: 10.1128/EC.00114-07. Epub 2007 Sep 7. Eukaryot Cell. 2007. PMID: 17827348 Free PMC article.
-
Sir2 regulation by nicotinamide results from switching between base exchange and deacetylation chemistry.Biochemistry. 2003 Aug 12;42(31):9249-56. doi: 10.1021/bi034959l. Biochemistry. 2003. PMID: 12899610
-
SIR2: the biochemical mechanism of NAD(+)-dependent protein deacetylation and ADP-ribosyl enzyme intermediates.Curr Med Chem. 2004 Apr;11(7):807-26. doi: 10.2174/0929867043455675. Curr Med Chem. 2004. PMID: 15078167 Review.
-
Sirtuin chemical mechanisms.Biochim Biophys Acta. 2010 Aug;1804(8):1591-603. doi: 10.1016/j.bbapap.2010.01.021. Epub 2010 Feb 2. Biochim Biophys Acta. 2010. PMID: 20132909 Free PMC article. Review.
Cited by
-
Transition state of ADP-ribosylation of acetyllysine catalyzed by Archaeoglobus fulgidus Sir2 determined by kinetic isotope effects and computational approaches.J Am Chem Soc. 2010 Sep 8;132(35):12286-98. doi: 10.1021/ja910342d. J Am Chem Soc. 2010. PMID: 20718419 Free PMC article.
-
Azalysine analogues as probes for protein lysine deacetylation and demethylation.J Am Chem Soc. 2012 Mar 21;134(11):5138-48. doi: 10.1021/ja209574z. Epub 2012 Mar 12. J Am Chem Soc. 2012. PMID: 22352831 Free PMC article.
-
The diversity of histone versus nonhistone sirtuin substrates.Genes Cancer. 2013 Mar;4(3-4):148-63. doi: 10.1177/1947601913483767. Genes Cancer. 2013. PMID: 24020006 Free PMC article.
-
Structural and functional analysis of human SIRT1.J Mol Biol. 2014 Feb 6;426(3):526-41. doi: 10.1016/j.jmb.2013.10.009. Epub 2013 Oct 10. J Mol Biol. 2014. PMID: 24120939 Free PMC article.
-
Side chain specificity of ADP-ribosylation by a sirtuin.FEBS J. 2009 Dec;276(23):7159-76. doi: 10.1111/j.1742-4658.2009.07427.x. Epub 2009 Nov 6. FEBS J. 2009. PMID: 19895577 Free PMC article.
References
-
- Sauve AA, Wolberger C, Schramm VL, Boeke JD. The biochemistry of sirtuins. Annu Rev Biochem. 2006;75:435–465. - PubMed
-
- Blander G, Guarente L. The Sir2 family of protein deacetylases. Annu Rev Biochem. 2004;73:417–435. - PubMed
-
- Guarente L. Sirtuins as potential targets for metabolic syndrome. Nature. 2006;444:868–874. - PubMed
-
- Haigis MC, Guarente LP. Mammalian sirtuins--emerging roles in physiology, aging, and calorie restriction. Genes Dev. 2006;20:2913–2921. - PubMed
-
- Liszt G, Ford E, Kurtev M, Guarente L. Mouse Sir2 homolog SIRT6 is a nuclear ADP-ribosyltransferase. J Biol Chem. 2005;280:21313–21320. - PubMed
Publication types
MeSH terms
Substances
Grants and funding
LinkOut - more resources
Full Text Sources
Molecular Biology Databases