The actin cytoskeleton of kidney podocytes is a direct target of the antiproteinuric effect of cyclosporine A
- PMID: 18724379
- PMCID: PMC4109287
- DOI: 10.1038/nm.1857
The actin cytoskeleton of kidney podocytes is a direct target of the antiproteinuric effect of cyclosporine A
Abstract
The immunosuppressive action of the calcineurin inhibitor cyclosporine A (CsA) stems from the inhibition of nuclear factor of activated T cells (NFAT) signaling in T cells. CsA is also used for the treatment of proteinuric kidney diseases. As it stands, the antiproteinuric effect of CsA is attributed to its immunosuppressive action. Here we show that the beneficial effect of CsA on proteinuria is not dependent on NFAT inhibition in T cells, but rather results from the stabilization of the actin cytoskeleton in kidney podocytes. CsA blocks the calcineurin-mediated dephosphorylation of synaptopodin, a regulator of Rho GTPases in podocytes, thereby preserving the phosphorylation-dependent synaptopodin-14-3-3 beta interaction. Preservation of this interaction, in turn, protects synaptopodin from cathepsin L-mediated degradation. These results represent a new view of calcineurin signaling and shed further light on the treatment of proteinuric kidney diseases. Novel calcineurin substrates such as synaptopodin may provide promising starting points for antiproteinuric drugs that avoid the serious side effects of long-term CsA treatment.
Figures
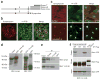
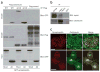
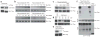
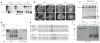
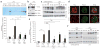
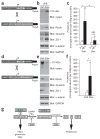
Comment in
-
Is the LPS-mediated proteinuria mouse model relevant to human kidney disease?Nat Med. 2009 Feb;15(2):133; author reply 133-4. doi: 10.1038/nm0209-133a. Nat Med. 2009. PMID: 19197281 No abstract available.
Similar articles
-
Proteinuria and immunity--an overstated relationship?N Engl J Med. 2008 Dec 4;359(23):2492-4. doi: 10.1056/NEJMcibr0806881. N Engl J Med. 2008. PMID: 19052132 No abstract available.
-
Cyclosporine A protects podocytes by regulating WAVE1 phosphorylation.Sci Rep. 2015 Dec 4;5:17694. doi: 10.1038/srep17694. Sci Rep. 2015. PMID: 26634693 Free PMC article.
-
Is the antiproteinuric effect of cyclosporine a independent of its immunosuppressive function in T cells?Int J Nephrol. 2012;2012:809456. doi: 10.1155/2012/809456. Epub 2012 Jun 13. Int J Nephrol. 2012. PMID: 22778954 Free PMC article.
-
Inhibitors of the calcineurin/NFAT pathway.Curr Med Chem. 2004 Apr;11(8):997-1007. doi: 10.2174/0929867043455576. Curr Med Chem. 2004. PMID: 15078162 Review.
-
Proteinuria: an enzymatic disease of the podocyte?Kidney Int. 2010 Apr;77(7):571-80. doi: 10.1038/ki.2009.424. Epub 2009 Nov 18. Kidney Int. 2010. PMID: 19924101 Free PMC article. Review.
Cited by
-
The role of the podocyte in albumin filtration.Nat Rev Nephrol. 2013 Jun;9(6):328-36. doi: 10.1038/nrneph.2013.78. Epub 2013 Apr 23. Nat Rev Nephrol. 2013. PMID: 23609563 Review.
-
Low- and high-molecular-weight urinary proteins as predictors of response to rituximab in patients with membranous nephropathy: a prospective study.Nephrol Dial Transplant. 2013 Jan;28(1):137-46. doi: 10.1093/ndt/gfs379. Epub 2012 Sep 17. Nephrol Dial Transplant. 2013. PMID: 22987142 Free PMC article.
-
Drug discovery in focal and segmental glomerulosclerosis.Kidney Int. 2016 Jun;89(6):1211-20. doi: 10.1016/j.kint.2015.12.058. Epub 2016 Apr 23. Kidney Int. 2016. PMID: 27165834 Free PMC article. Review.
-
Pharmacological targeting of actin-dependent dynamin oligomerization ameliorates chronic kidney disease in diverse animal models.Nat Med. 2015 Jun;21(6):601-9. doi: 10.1038/nm.3843. Epub 2015 May 11. Nat Med. 2015. PMID: 25962121 Free PMC article.
-
Tacrolimus Protects Podocytes from Injury in Lupus Nephritis Partly by Stabilizing the Cytoskeleton and Inhibiting Podocyte Apoptosis.PLoS One. 2015 Jul 10;10(7):e0132724. doi: 10.1371/journal.pone.0132724. eCollection 2015. PLoS One. 2015. PMID: 26161538 Free PMC article.
References
-
- Somlo S, Mundel P. Getting a foothold in nephrotic syndrome. Nat Genet. 2000;24:333–335. - PubMed
-
- Tryggvason K, Patrakka J, Wartiovaara J. Hereditary proteinuria syndromes and mechanisms of proteinuria. N Engl J Med. 2006;354:1387–1401. - PubMed
-
- Tryggvason K, Pikkarainen T, Patrakka J. Nck links nephrin to actin in kidney podocytes. Cell. 2006;125:221–224. - PubMed
-
- Faul C, Asanuma K, Yanagida-Asanuma E, Kim K, Mundel P. Actin up: regulation of podocyte structure and function by components of the actin cytoskeleton. Trends Cell Biol. 2007;17:428–437. - PubMed
Publication types
MeSH terms
Substances
Grants and funding
LinkOut - more resources
Full Text Sources
Other Literature Sources
Research Materials