A complex RNA motif defined by three discontinuous 5-nucleotide-long strands is essential for Flavivirus RNA replication
- PMID: 18669441
- PMCID: PMC2525960
- DOI: 10.1261/rna.993608
A complex RNA motif defined by three discontinuous 5-nucleotide-long strands is essential for Flavivirus RNA replication
Abstract
Tertiary or higher-order RNA motifs that regulate replication of positive-strand RNA viruses are as yet poorly understood. Using Japanese encephalitis virus (JEV), we now show that a key element in JEV RNA replication is a complex RNA motif that includes a string of three discontinuous complementary sequences (TDCS). The TDCS consists of three 5-nt-long strands, the left (L) strand upstream of the translation initiator AUG adjacent to the 5'-end of the genome, and the middle (M) and right (R) strands corresponding to the base of the Flavivirus-conserved 3' stem-loop structure near the 3'-end of the RNA. The three strands are arranged in an antiparallel configuration, with two sets of base-pairing interactions creating L-M and M-R duplexes. Disrupting either or both of these duplex regions of TDCS completely abolished RNA replication, whereas reconstructing both duplex regions, albeit with mutated sequences, fully restored RNA replication. Modeling of replication-competent genomes recovered from a large pool of pseudorevertants originating from six replication-incompetent TDCS mutants suggests that both duplex base-pairing potentials of TDCS are required for RNA replication. In all cases, acquisition of novel sequences within the 3'M-R duplex facilitated a long-range RNA-RNA interaction of its 3'M strand with either the authentic 5'L strand or its alternative (invariably located upstream of the 5' initiator), thereby restoring replicability. We also found that a TDCS homolog is conserved in other flaviviruses. These data suggest that two duplex base-pairings defined by the TDCS play an essential regulatory role in a key step(s) of Flavivirus RNA replication.
Figures
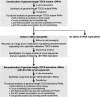
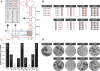
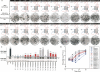
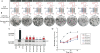
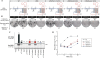
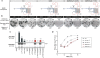
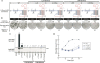
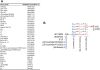
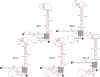
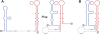
Similar articles
-
3' cis-acting elements that contribute to the competence and efficiency of Japanese encephalitis virus genome replication: functional importance of sequence duplications, deletions, and substitutions.J Virol. 2009 Aug;83(16):7909-30. doi: 10.1128/JVI.02541-08. Epub 2009 Jun 3. J Virol. 2009. PMID: 19494005 Free PMC article.
-
Inhibition of Japanese encephalitis virus replication by peptide nucleic acids targeting cis-acting elements on the plus- and minus-strands of viral RNA.Antiviral Res. 2009 Jun;82(3):122-33. doi: 10.1016/j.antiviral.2009.02.187. Epub 2009 Feb 20. Antiviral Res. 2009. PMID: 19428603
-
Essential role of cyclization sequences in flavivirus RNA replication.J Virol. 2001 Jul;75(14):6719-28. doi: 10.1128/JVI.75.14.6719-6728.2001. J Virol. 2001. PMID: 11413342 Free PMC article.
-
Genome cyclization as strategy for flavivirus RNA replication.Virus Res. 2009 Feb;139(2):230-9. doi: 10.1016/j.virusres.2008.07.016. Epub 2008 Sep 9. Virus Res. 2009. PMID: 18703097 Free PMC article. Review.
-
Functions of the 3' and 5' genome RNA regions of members of the genus Flavivirus.Virus Res. 2015 Aug 3;206:108-19. doi: 10.1016/j.virusres.2015.02.006. Epub 2015 Feb 13. Virus Res. 2015. PMID: 25683510 Free PMC article. Review.
Cited by
-
RNA-RNA and RNA-protein interactions in coronavirus replication and transcription.RNA Biol. 2011 Mar-Apr;8(2):237-48. doi: 10.4161/rna.8.2.14991. Epub 2011 Mar 1. RNA Biol. 2011. PMID: 21378501 Free PMC article. Review.
-
Bacterial Artificial Chromosomes: A Functional Genomics Tool for the Study of Positive-strand RNA Viruses.J Vis Exp. 2015 Dec 29;(106):e53164. doi: 10.3791/53164. J Vis Exp. 2015. PMID: 26780115 Free PMC article.
-
A balance between circular and linear forms of the dengue virus genome is crucial for viral replication.RNA. 2010 Dec;16(12):2325-35. doi: 10.1261/rna.2120410. Epub 2010 Oct 27. RNA. 2010. PMID: 20980673 Free PMC article.
-
Functional Information Stored in the Conserved Structural RNA Domains of Flavivirus Genomes.Front Microbiol. 2017 Apr 3;8:546. doi: 10.3389/fmicb.2017.00546. eCollection 2017. Front Microbiol. 2017. PMID: 28421048 Free PMC article. Review.
-
Profiling of viral proteins expressed from the genomic RNA of Japanese encephalitis virus using a panel of 15 region-specific polyclonal rabbit antisera: implications for viral gene expression.PLoS One. 2015 Apr 27;10(4):e0124318. doi: 10.1371/journal.pone.0124318. eCollection 2015. PLoS One. 2015. PMID: 25915765 Free PMC article.
References
Publication types
MeSH terms
Substances
LinkOut - more resources
Full Text Sources
Molecular Biology Databases
Research Materials