The dynamics of mammalian P body transport, assembly, and disassembly in vivo
- PMID: 18653466
- PMCID: PMC2555939
- DOI: 10.1091/mbc.e08-05-0513
The dynamics of mammalian P body transport, assembly, and disassembly in vivo
Abstract
Exported mRNAs are targeted for translation or can undergo degradation by several decay mechanisms. The 5'-->3' degradation machinery localizes to cytoplasmic P bodies (PBs). We followed the dynamic properties of PBs in vivo and investigated the mechanism by which PBs scan the cytoplasm. Using proteins of the decapping machinery, we asked whether PBs actively scan the cytoplasm or whether a diffusion-based mechanism is sufficient. Live-cell imaging showed that PBs were anchored mainly to microtubules. Quantitative single-particle tracking demonstrated that most PBs exhibited spatially confined motion dependent on microtubule motion, whereas stationary PB pairs were identified at the centrosome. Some PBs translocated in long-range movements on microtubules. PB mobility was compared with mitochondria, endoplasmic reticulum, peroxisomes, SMN bodies, and stress granules, and diffusion coefficients were calculated. Disruption of the microtubule network caused a significant reduction in PB mobility together with an induction of PB assembly. However, FRAP measurements showed that the dynamic flux of assembled PB components was not affected by such treatments. FRAP analysis showed that the decapping enzyme Dcp2 is a nondynamic PB core protein, whereas Dcp1 proteins continuously exchanged with the cytoplasm. This study reveals the mechanism of PB transport, and it demonstrates how PB assembly and disassembly integrate with the presence of an intact cytoskeleton.
Figures
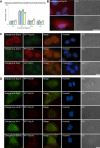
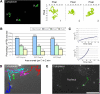
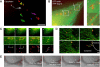
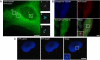
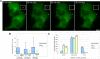
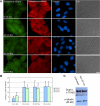
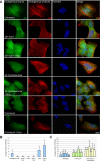
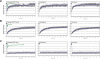
Similar articles
-
Intracellular trafficking and dynamics of P bodies.Prion. 2008 Oct-Dec;2(4):131-4. doi: 10.4161/pri.2.4.7773. Epub 2008 Oct 3. Prion. 2008. PMID: 19242093 Free PMC article. Review.
-
The control of mRNA decapping and P-body formation.Mol Cell. 2008 Dec 5;32(5):605-15. doi: 10.1016/j.molcel.2008.11.001. Mol Cell. 2008. PMID: 19061636 Free PMC article. Review.
-
Diffuse decapping enzyme DCP2 accumulates in DCP1 foci under heat stress in Arabidopsis thaliana.Plant Cell Physiol. 2015 Jan;56(1):107-15. doi: 10.1093/pcp/pcu151. Epub 2014 Oct 22. Plant Cell Physiol. 2015. PMID: 25339350
-
The sudden recruitment of gamma-tubulin to the centrosome at the onset of mitosis and its dynamic exchange throughout the cell cycle, do not require microtubules.J Cell Biol. 1999 Aug 9;146(3):585-96. doi: 10.1083/jcb.146.3.585. J Cell Biol. 1999. PMID: 10444067 Free PMC article.
-
Microtubules govern stress granule mobility and dynamics.Biochim Biophys Acta. 2010 Mar;1803(3):361-71. doi: 10.1016/j.bbamcr.2009.12.004. Epub 2009 Dec 28. Biochim Biophys Acta. 2010. PMID: 20036288
Cited by
-
Dynein and kinesin regulate stress-granule and P-body dynamics.J Cell Sci. 2009 Nov 1;122(Pt 21):3973-82. doi: 10.1242/jcs.051383. Epub 2009 Oct 13. J Cell Sci. 2009. PMID: 19825938 Free PMC article.
-
Drosophila Doublefault protein coordinates multiple events during male meiosis by controlling mRNA translation.Development. 2019 Nov 18;146(22):dev183053. doi: 10.1242/dev.183053. Development. 2019. PMID: 31645358 Free PMC article.
-
A distinct P-body-like granule is induced in response to the disruption of microtubule integrity in Saccharomyces cerevisiae.Genetics. 2022 Aug 30;222(1):iyac105. doi: 10.1093/genetics/iyac105. Genetics. 2022. PMID: 35876801 Free PMC article.
-
mRNA decapping enzyme 1a (Dcp1a)-induced translational arrest through protein kinase R (PKR) activation requires the N-terminal enabled vasodilator-stimulated protein homology 1 (EVH1) domain.J Biol Chem. 2014 Feb 14;289(7):3936-49. doi: 10.1074/jbc.M113.518191. Epub 2013 Dec 31. J Biol Chem. 2014. PMID: 24382890 Free PMC article.
-
Transforming growth factor β regulates P-body formation through induction of the mRNA decay factor tristetraprolin.Mol Cell Biol. 2014 Jan;34(2):180-95. doi: 10.1128/MCB.01020-13. Epub 2013 Nov 4. Mol Cell Biol. 2014. PMID: 24190969 Free PMC article.
References
-
- Beelman C. A., Stevens A., Caponigro G., LaGrandeur T. E., Hatfield L., Fortner D. M., Parker R. An essential component of the decapping enzyme required for normal rates of mRNA turnover. Nature. 1996;382:642–646. - PubMed
Publication types
MeSH terms
Substances
LinkOut - more resources
Full Text Sources
Other Literature Sources
Miscellaneous