Protein structure protection commits gene expression patterns
- PMID: 18606003
- PMCID: PMC2530859
- DOI: 10.1186/gb-2008-9-7-r107
Protein structure protection commits gene expression patterns
Abstract
Background: Gene co-expressions often determine module-defining spatial and temporal concurrences of proteins. Yet, little effort has been devoted to tracing coordinating signals for expression correlations to the three-dimensional structures of gene products.
Results: We performed a global structure-based analysis of the yeast and human proteomes and contrasted this information against their respective transcriptome organizations obtained from comprehensive microarray data. We show that protein vulnerability quantifies dosage sensitivity for metabolic adaptation phases and tissue-specific patterns of mRNA expression, determining the extent of co-expression similarity of binding partners. The role of protein intrinsic disorder in transcriptome organization is also delineated by interrelating vulnerability, disorder propensity and co-expression patterns. Extremely vulnerable human proteins are shown to be subject to severe post-transcriptional regulation of their expression through significant micro-RNA targeting, making mRNA levels poor surrogates for protein-expression levels. By contrast, in yeast the expression of extremely under-wrapped proteins is likely regulated through protein aggregation. Thus, the 85 most vulnerable proteins in yeast include the five confirmed prions, while in human, the genes encoding extremely vulnerable proteins are predicted to be targeted by microRNAs. Hence, in both vastly different organisms protein vulnerability emerges as a structure-encoded signal for post-transcriptional regulation.
Conclusion: Vulnerability of protein structure and the concurrent need to maintain structural integrity are shown to quantify dosage sensitivity, compelling gene expression patterns across tissue types and temporal adaptation phases in a quantifiable manner. Extremely vulnerable proteins impose additional constraints on gene expression: They are subject to high levels of regulation at the post-transcriptional level.
Figures
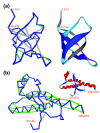
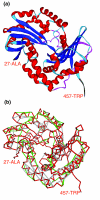
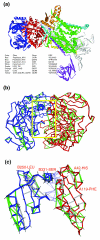
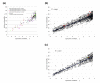
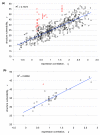
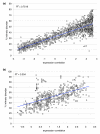
Similar articles
-
Evolutionary Conservation and Diversification of Puf RNA Binding Proteins and Their mRNA Targets.PLoS Biol. 2015 Nov 20;13(11):e1002307. doi: 10.1371/journal.pbio.1002307. eCollection 2015. PLoS Biol. 2015. PMID: 26587879 Free PMC article.
-
Protein under-wrapping causes dosage sensitivity and decreases gene duplicability.PLoS Genet. 2008 Jan;4(1):e11. doi: 10.1371/journal.pgen.0040011. Epub 2007 Dec 13. PLoS Genet. 2008. PMID: 18208334 Free PMC article.
-
Analysis of the yeast transcriptome with structural and functional categories: characterizing highly expressed proteins.Nucleic Acids Res. 2000 Mar 15;28(6):1481-8. doi: 10.1093/nar/28.6.1481. Nucleic Acids Res. 2000. PMID: 10684945 Free PMC article.
-
Protein wrapping: a molecular marker for association, aggregation and drug design.Chem Soc Rev. 2008 Nov;37(11):2373-82. doi: 10.1039/b804150b. Epub 2008 Sep 15. Chem Soc Rev. 2008. PMID: 18949110 Review.
-
Engineering Aspects of Olfaction.In: Persaud KC, Marco S, Gutiérrez-Gálvez A, editors. Neuromorphic Olfaction. Boca Raton (FL): CRC Press/Taylor & Francis; 2013. Chapter 1. In: Persaud KC, Marco S, Gutiérrez-Gálvez A, editors. Neuromorphic Olfaction. Boca Raton (FL): CRC Press/Taylor & Francis; 2013. Chapter 1. PMID: 26042329 Free Books & Documents. Review.
Cited by
-
A decade and a half of protein intrinsic disorder: biology still waits for physics.Protein Sci. 2013 Jun;22(6):693-724. doi: 10.1002/pro.2261. Epub 2013 Apr 29. Protein Sci. 2013. PMID: 23553817 Free PMC article. Review.
-
New insights into prion biology from the novel [SWI+] system.Prion. 2008 Oct-Dec;2(4):141-4. doi: 10.4161/pri.2.4.8069. Prion. 2008. PMID: 19256027 Free PMC article. Review.
-
Protein intrinsic disorder as a flexible armor and a weapon of HIV-1.Cell Mol Life Sci. 2012 Apr;69(8):1211-59. doi: 10.1007/s00018-011-0859-3. Epub 2011 Oct 28. Cell Mol Life Sci. 2012. PMID: 22033837 Free PMC article. Review.
-
A generally applicable translational strategy identifies S100A4 as a candidate gene in allergy.Sci Transl Med. 2014 Jan 8;6(218):218ra4. doi: 10.1126/scitranslmed.3007410. Sci Transl Med. 2014. PMID: 24401939 Free PMC article.
-
Insights into the regulation of intrinsically disordered proteins in the human proteome by analyzing sequence and gene expression data.Genome Biol. 2009;10(5):R50. doi: 10.1186/gb-2009-10-5-r50. Epub 2009 May 11. Genome Biol. 2009. PMID: 19432952 Free PMC article.
References
-
- Uetz P, Giot L, Cagney G, Mansfield TA, Judson RS, Knight JR, Lockshon D, Narayan V, Srinivasan M, Pochart P, Qureshi-Emili A, Li Y, Godwin B, Conover D, Kalbfleisch D, Vijayadamodar G, Yang M, Johnston M, Fields S, Rothberg JM. A comprehensive analysis of protein-protein interactions in Saccharomyces cerevisiae. Nature. 2000;403:623–627. - PubMed
-
- Gavin AC, Aloy P, Grandi P, Krause R, Boesche M, Marzioch M, Rau C, Jensen LJ, Bastuck S, Dümpelfeld B, Edelmann A, Heurtier MA, Hoffman V, Hoefert C, Klein K, Hudak M, Michon AM, Schelder M, Schirle M, Remor M, Rudi T, Hooper S, Bauer A, Bouwmeester T, Casari G, Drewes G, Neubauer G, Rick JM, Kuster B, Bork P, et al. Proteome survey reveals modularity of the yeast cell machinery. Nature. 2006;440:631–636. - PubMed
-
- Hartwell LH, Hopfield JJ, Leibler S, Murray AW. From molecular to modular cell biology. Nature. 1999;402:C47–C52. - PubMed
-
- Ravasz E, Somera AL, Mongru DA, Oltvai ZN, Barabasi AL. Hierarchical organization of modularity in metabolic networks. Science. 2002;297:1551–1555. - PubMed
-
- Ge H, Liu Z, Church GM, Vidal M. Correlation between transcriptome and interactome mapping data from Saccharomyces cerevisiae. Nat Genet. 2001;29:482–486. - PubMed
Publication types
MeSH terms
Substances
Grants and funding
LinkOut - more resources
Full Text Sources
Molecular Biology Databases