Hemopexin prevents endothelial damage and liver congestion in a mouse model of heme overload
- PMID: 18556779
- PMCID: PMC2438305
- DOI: 10.2353/ajpath.2008.071130
Hemopexin prevents endothelial damage and liver congestion in a mouse model of heme overload
Abstract
Intravascular hemolysis results in the release of massive amounts of hemoglobin and heme into plasma, where they are rapidly bound by haptoglobin and hemopexin, respectively. Data from haptoglobin and hemopexin knockout mice have shown that both proteins protect from renal damage after phenylhydrazine-induced hemolysis, whereas double-mutant mice were especially prone to liver damage. However, the specific role of hemopexin remains elusive because of the difficulty in discriminating between hemoglobin and heme recovery. To study the specific role of hemopexin in intravascular hemolysis, we established a mouse model of heme overload. Under these conditions, both endothelial activation and vascular permeability were significantly higher in hemopexin-null mice compared with wild-type controls. Vascular permeability was particularly altered in the liver, where congestion in the centrolobular area was believed to be associated with oxidative stress and inflammation. Liver damage in hemopexin- null mice may be prevented by induction of heme oxygenase-1 before heme overload. Furthermore, heme-treated hemopexin-null mice exhibited hyperbilirubinemia, prolonged heme oxygenase-1 expression, excessive heme metabolism, and lack of H-ferritin induction in the liver compared with heme-treated wild-type controls. Moreover, these mutant mice metabolize an excess of heme in the kidney. These studies highlight the importance of hemopexin in heme detoxification, thus suggesting that drugs mimicking hemopexin activity might be useful to prevent endothelial damage in patients suffering from hemolytic disorders.
Figures
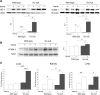
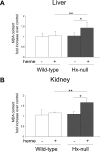
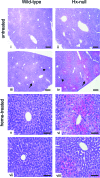
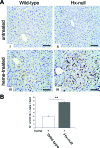
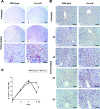
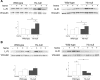
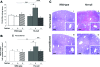
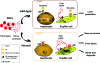
Similar articles
-
Haptoglobin and hemopexin inhibit vaso-occlusion and inflammation in murine sickle cell disease: Role of heme oxygenase-1 induction.PLoS One. 2018 Apr 25;13(4):e0196455. doi: 10.1371/journal.pone.0196455. eCollection 2018. PLoS One. 2018. PMID: 29694434 Free PMC article.
-
Hemopexin therapy improves cardiovascular function by preventing heme-induced endothelial toxicity in mouse models of hemolytic diseases.Circulation. 2013 Mar 26;127(12):1317-29. doi: 10.1161/CIRCULATIONAHA.112.130179. Epub 2013 Feb 27. Circulation. 2013. PMID: 23446829
-
Enhanced splenomegaly and severe liver inflammation in haptoglobin/hemopexin double-null mice after acute hemolysis.Blood. 2002 Dec 1;100(12):4201-8. doi: 10.1182/blood-2002-04-1270. Epub 2002 Jul 25. Blood. 2002. PMID: 12393471
-
Therapeutic approaches to limit hemolysis-driven endothelial dysfunction: scavenging free heme to preserve vasculature homeostasis.Oxid Med Cell Longev. 2013;2013:396527. doi: 10.1155/2013/396527. Epub 2013 May 27. Oxid Med Cell Longev. 2013. PMID: 23781294 Free PMC article. Review.
-
The Worst Things in Life are Free: The Role of Free Heme in Sickle Cell Disease.Front Immunol. 2021 Jan 27;11:561917. doi: 10.3389/fimmu.2020.561917. eCollection 2020. Front Immunol. 2021. PMID: 33584641 Free PMC article. Review.
Cited by
-
Multifaceted Role of Heme during Severe Plasmodium falciparum Infections in India.Infect Immun. 2015 Oct;83(10):3793-9. doi: 10.1128/IAI.00531-15. Epub 2015 Jul 13. Infect Immun. 2015. PMID: 26169278 Free PMC article.
-
Haptoglobin and hemopexin inhibit vaso-occlusion and inflammation in murine sickle cell disease: Role of heme oxygenase-1 induction.PLoS One. 2018 Apr 25;13(4):e0196455. doi: 10.1371/journal.pone.0196455. eCollection 2018. PLoS One. 2018. PMID: 29694434 Free PMC article.
-
HO‑1 knockdown upregulates the expression of VCAM‑1 to induce neutrophil recruitment during renal ischemia‑reperfusion injury.Int J Mol Med. 2021 Oct;48(4):185. doi: 10.3892/ijmm.2021.5018. Epub 2021 Aug 9. Int J Mol Med. 2021. PMID: 34368855 Free PMC article.
-
Data demonstrating the anti-oxidant role of hemopexin in the heart.Data Brief. 2017 May 13;13:69-76. doi: 10.1016/j.dib.2017.05.026. eCollection 2017 Aug. Data Brief. 2017. PMID: 28560284 Free PMC article.
-
Heme-Mediated Induction of CXCL10 and Depletion of CD34+ Progenitor Cells Is Toll-Like Receptor 4 Dependent.PLoS One. 2015 Nov 10;10(11):e0142328. doi: 10.1371/journal.pone.0142328. eCollection 2015. PLoS One. 2015. PMID: 26555697 Free PMC article.
References
-
- Kumar S, Bandyopadhyay U. Free heme toxicity and its detoxification systems in human. Toxicol Lett. 2005;157:175–188. - PubMed
-
- Wagener FA, Volk HD, Willis D, Abraham NG, Soares MP, Adema GJ, Figdor CG. Different faces of the heme-heme oxygenase system in inflammation. Pharmacol Rev. 2003;55:551–571. - PubMed
-
- Balla J, Balla G, Jeney V, Kakuk G, Jacob HS, Vercellotti GM. Ferriporphyrins and endothelium: a 2-edged sword-promotion of oxidation and induction of cytoprotectants. Blood. 2000;95:3442–3450. - PubMed
-
- Jeney V, Balla J, Yachie A, Varga Z, Vercellotti GM, Eaton JW, Balla G. Pro-oxidant and cytotoxic effects of circulating heme. Blood. 2002;100:879–887. - PubMed
-
- Belcher JD, Bryant CJ, Nguyen J, Bowlin PR, Kielbik MC, Bischof JC, Hebbel RP, Vercellotti GM. Transgenic sickle mice have vascular inflammation. Blood. 2003;101:3953–3959. - PubMed
MeSH terms
Substances
Grants and funding
LinkOut - more resources
Full Text Sources
Other Literature Sources
Medical
Molecular Biology Databases