Disorders of lysosome-related organelle biogenesis: clinical and molecular genetics
- PMID: 18544035
- PMCID: PMC2755194
- DOI: 10.1146/annurev.genom.9.081307.164303
Disorders of lysosome-related organelle biogenesis: clinical and molecular genetics
Abstract
Lysosome-related organelles (LROs) are a heterogeneous group of vesicles that share various features with lysosomes, but are distinct in function, morphology, and composition. The biogenesis of LROs employs a common machinery, and genetic defects in this machinery can affect all LROs or only an individual LRO, resulting in a variety of clinical features. In this review, we discuss the main components of LRO biogenesis. We also summarize the function, composition, and resident cell types of the major LROs. Finally, we describe the clinical characteristics of the major human LRO disorders.
Figures
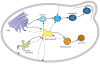
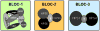
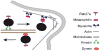
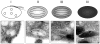
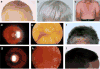
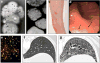
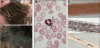
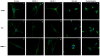
Similar articles
-
Lysosome-related organelles.FASEB J. 2000 Jul;14(10):1265-78. doi: 10.1096/fj.14.10.1265. FASEB J. 2000. PMID: 10877819 Review.
-
Insights into the biogenesis of lysosome-related organelles from the study of the Hermansky-Pudlak syndrome.Ann N Y Acad Sci. 2004 Dec;1038:103-14. doi: 10.1196/annals.1315.018. Ann N Y Acad Sci. 2004. PMID: 15838104
-
Genetic regulation of Caenorhabditis elegans lysosome related organelle function.PLoS Genet. 2013 Oct;9(10):e1003908. doi: 10.1371/journal.pgen.1003908. Epub 2013 Oct 24. PLoS Genet. 2013. PMID: 24204312 Free PMC article.
-
Hermansky-Pudlak syndrome and Chediak-Higashi syndrome: disorders of vesicle formation and trafficking.Thromb Haemost. 2001 Jul;86(1):233-45. Thromb Haemost. 2001. PMID: 11487012 Review.
-
Hermansky-Pudlak syndrome: pigmentary and non-pigmentary defects and their pathogenesis.Pigment Cell Melanoma Res. 2013 Mar;26(2):176-92. doi: 10.1111/pcmr.12051. Epub 2012 Dec 31. Pigment Cell Melanoma Res. 2013. PMID: 23171219 Review.
Cited by
-
Mouse RC/BTB2, a member of the RCC1 superfamily, localizes to spermatid acrosomal vesicles.PLoS One. 2012;7(6):e39846. doi: 10.1371/journal.pone.0039846. Epub 2012 Jun 29. PLoS One. 2012. PMID: 22768142 Free PMC article.
-
The WASH complex, an endosomal Arp2/3 activator, interacts with the Hermansky-Pudlak syndrome complex BLOC-1 and its cargo phosphatidylinositol-4-kinase type IIα.Mol Biol Cell. 2013 Jul;24(14):2269-84. doi: 10.1091/mbc.E13-02-0088. Epub 2013 May 15. Mol Biol Cell. 2013. PMID: 23676666 Free PMC article.
-
Acrosome biogenesis: Revisiting old questions to yield new insights.Spermatogenesis. 2011 Apr;1(2):95-98. doi: 10.4161/spmg.1.2.16820. Spermatogenesis. 2011. PMID: 22319656 Free PMC article.
-
Drosophila mauve mutants reveal a role of LYST homologs late in the maturation of phagosomes and autophagosomes.Traffic. 2012 Dec;13(12):1680-92. doi: 10.1111/tra.12005. Epub 2012 Sep 20. Traffic. 2012. PMID: 22934826 Free PMC article.
-
Assembly of the biogenesis of lysosome-related organelles complex-3 (BLOC-3) and its interaction with Rab9.J Biol Chem. 2010 Mar 5;285(10):7794-804. doi: 10.1074/jbc.M109.069088. Epub 2010 Jan 4. J Biol Chem. 2010. PMID: 20048159 Free PMC article.
References
-
- Ali B, Seabra M. Targeting of Rab GTPases to cellular membranes. Biochem Soc Trans. 2005;33:652–6. - PubMed
-
- Anderson PD, Huizing M, Claassen DA, White J, Gahl WA. Hermansky-Pudlak syndrome type 4 (HPS-4): clinical and molecular characteristics. Hum Genet. 2003;113:10–7. - PubMed
-
- Anikster Y, Huizing M, White J, Shevchenko YO, Fitzpatrick DL, et al. Mutation of a new gene causes a unique form of Hermansky-Pudlak syndrome in a genetic isolate of central Puerto Rico. Nat Genet. 2001;28:376–80. - PubMed
-
- Barral DC, Seabra MC. The melanosome as a model to study organelle motility in mammals. Pigment Cell Res. 2004;17:111–8. - PubMed
-
- Berson JF, Theos AC, Harper DC, Tenza D, Raposo G, Marks MS. Proprotein convertase cleavage liberates a fibrillogenic fragment of a resident glycoprotein to initiate melanosome biogenesis. J Cell Biol. 2003;161:521–33. This manuscript describes MVBs as intermediates in the generation of stage II melanosomes, and it shows that PMEL17 is the main component of the intralumenal fibrils of stage II melanosomes. These fibrils are generated by cleavage of PMEL17 in a post-Golgi compartment. - PMC - PubMed
Publication types
MeSH terms
Grants and funding
LinkOut - more resources
Full Text Sources
Other Literature Sources
Medical