Yeast translational response to high salinity: global analysis reveals regulation at multiple levels
- PMID: 18495938
- PMCID: PMC2441982
- DOI: 10.1261/rna.864908
Yeast translational response to high salinity: global analysis reveals regulation at multiple levels
Abstract
Genome-wide studies of steady-state mRNA levels revealed common principles underlying transcriptional changes in response to external stimuli. To uncover principles that govern other stages of the gene-expression response, we analyzed the translational response and its coordination with transcriptome changes following exposure to severe stress. Yeast cells were grown for 1 h in medium containing 1 M NaCl, which elicits a maximal but transient translation inhibition, and nonpolysomal or polysomal mRNA pools were subjected to DNA-microarray analyses. We observed a strong repression in polysomal association for most mRNAs, with no simple correlation with the changes in transcript levels. This led to an apparent accumulation of many mRNAs as a nontranslating pool, presumably waiting for recovery from the stress. However, some mRNAs demonstrated a correlated change in their polysomal association and their transcript levels (i.e., potentiation). This group was enriched with targets of the transcription factors Msn2/Msn4, and the translational induction of several tested mRNAs was diminished in an Msn2/Msn4 deletion strain. Genome-wide analysis of a strain lacking the high salinity response kinase Hog1p revealed that the group of translationally affected genes is significantly enriched with motifs that were shown to be associated with the ARE-binding protein Pub1. Since a relatively small number of genes was affected by Hog1p deletion, additional signaling pathways are likely to be involved in coordinating the translational response to severe salinity stress.
Figures
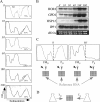
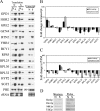
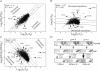
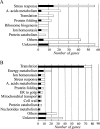
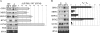
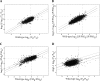
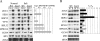
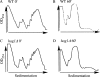
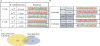
Similar articles
-
The HOG pathway dictates the short-term translational response after hyperosmotic shock.Mol Biol Cell. 2010 Sep 1;21(17):3080-92. doi: 10.1091/mbc.E10-01-0006. Epub 2010 Jun 29. Mol Biol Cell. 2010. PMID: 20587780 Free PMC article.
-
Global and specific translational regulation in the genomic response of Saccharomyces cerevisiae to a rapid transfer from a fermentable to a nonfermentable carbon source.Mol Cell Biol. 2001 Feb;21(3):916-27. doi: 10.1128/MCB.21.3.916-927.2001. Mol Cell Biol. 2001. PMID: 11154278 Free PMC article.
-
The Saccharomyces cerevisiae Hot1p regulated gene YHR087W (HGI1) has a role in translation upon high glucose concentration stress.BMC Mol Biol. 2012 Jun 21;13:19. doi: 10.1186/1471-2199-13-19. BMC Mol Biol. 2012. PMID: 22720784 Free PMC article.
-
Genome-wide analyses of early translational responses to elevated temperature and high salinity in Arabidopsis thaliana.Plant Cell Physiol. 2010 Mar;51(3):448-62. doi: 10.1093/pcp/pcq010. Epub 2010 Jan 19. Plant Cell Physiol. 2010. PMID: 20089509
-
Translational regulation in response to stress in Saccharomyces cerevisiae.Yeast. 2019 Jan;36(1):5-21. doi: 10.1002/yea.3349. Epub 2018 Sep 3. Yeast. 2019. PMID: 30019452 Free PMC article. Review.
Cited by
-
Mechanism and Regulation of Protein Synthesis in Saccharomyces cerevisiae.Genetics. 2016 May;203(1):65-107. doi: 10.1534/genetics.115.186221. Genetics. 2016. PMID: 27183566 Free PMC article. Review.
-
Multiple levels of gene regulation mediate differentiation of the intracellular pathogen Leishmania.FASEB J. 2011 Feb;25(2):515-25. doi: 10.1096/fj.10-157529. Epub 2010 Oct 15. FASEB J. 2011. PMID: 20952481 Free PMC article.
-
Yeast mRNA cap-binding protein Cbc1/Sto1 is necessary for the rapid reprogramming of translation after hyperosmotic shock.Mol Biol Cell. 2012 Jan;23(1):137-50. doi: 10.1091/mbc.E11-05-0419. Epub 2011 Nov 9. Mol Biol Cell. 2012. PMID: 22072789 Free PMC article.
-
Preferential Ribosome Loading on the Stress-Upregulated mRNA Pool Shapes the Selective Translation under Stress Conditions.Plants (Basel). 2021 Feb 5;10(2):304. doi: 10.3390/plants10020304. Plants (Basel). 2021. PMID: 33562590 Free PMC article.
-
Widespread translational control contributes to the regulation of Arabidopsis photomorphogenesis.Mol Syst Biol. 2012 Jan 17;8:566. doi: 10.1038/msb.2011.97. Mol Syst Biol. 2012. PMID: 22252389 Free PMC article.
References
-
- Albertyn J., Hohmann S., Thevelein J.M., Prior B.A. GPD1, which encodes glycerol-3-phosphate dehydrogenase, is essential for growth under osmotic stress in Saccharomyces cerevisiae, and its expression is regulated by the high-osmolarity glycerol response pathway. Mol. Cell. Biol. 1994;14:4135–4144. - PMC - PubMed
Publication types
MeSH terms
Substances
LinkOut - more resources
Full Text Sources
Other Literature Sources
Molecular Biology Databases