Modification of intracellular membrane structures for virus replication
- PMID: 18414501
- PMCID: PMC7096853
- DOI: 10.1038/nrmicro1890
Modification of intracellular membrane structures for virus replication
Abstract
Viruses are intracellular parasites that use the host cell they infect to produce new infectious progeny. Distinct steps of the virus life cycle occur in association with the cytoskeleton or cytoplasmic membranes, which are often modified during infection. Plus-stranded RNA viruses induce membrane proliferations that support the replication of their genomes. Similarly, cytoplasmic replication of some DNA viruses occurs in association with modified cellular membranes. We describe how viruses modify intracellular membranes, highlight similarities between the structures that are induced by viruses of different families and discuss how these structures could be formed.
Figures
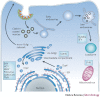
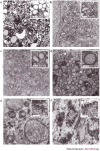
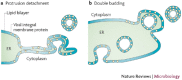
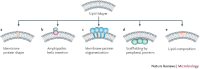
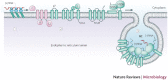
Similar articles
-
Alternate, virus-induced membrane rearrangements support positive-strand RNA virus genome replication.Proc Natl Acad Sci U S A. 2004 Aug 3;101(31):11263-8. doi: 10.1073/pnas.0404157101. Epub 2004 Jul 27. Proc Natl Acad Sci U S A. 2004. PMID: 15280537 Free PMC article.
-
Virus factories, double membrane vesicles and viroplasm generated in animal cells.Curr Opin Virol. 2011 Nov;1(5):381-7. doi: 10.1016/j.coviro.2011.09.008. Epub 2011 Oct 12. Curr Opin Virol. 2011. PMID: 22440839 Free PMC article. Review.
-
Ultrastructural Features of Membranous Replication Organelles Induced by Positive-Stranded RNA Viruses.Cells. 2021 Sep 13;10(9):2407. doi: 10.3390/cells10092407. Cells. 2021. PMID: 34572055 Free PMC article. Review.
-
COPII Vesicle Transport Is Required for Rotavirus NSP4 Interaction with the Autophagy Protein LC3 II and Trafficking to Viroplasms.J Virol. 2019 Dec 12;94(1):e01341-19. doi: 10.1128/JVI.01341-19. Print 2019 Dec 12. J Virol. 2019. PMID: 31597778 Free PMC article.
-
Virus-induced double-membrane vesicles.Cell Microbiol. 2015 Jan;17(1):45-50. doi: 10.1111/cmi.12372. Epub 2014 Oct 31. Cell Microbiol. 2015. PMID: 25287059 Free PMC article. Review.
Cited by
-
Ultrastructure of the replication sites of positive-strand RNA viruses.Virology. 2015 May;479-480:418-33. doi: 10.1016/j.virol.2015.02.029. Epub 2015 Mar 6. Virology. 2015. PMID: 25746936 Free PMC article. Review.
-
ACBD3-mediated recruitment of PI4KB to picornavirus RNA replication sites.EMBO J. 2012 Feb 1;31(3):754-66. doi: 10.1038/emboj.2011.429. Epub 2011 Nov 29. EMBO J. 2012. PMID: 22124328 Free PMC article.
-
The triglyceride-synthesizing enzyme diacylglycerol acyltransferase 2 modulates the formation of the hepatitis C virus replication organelle.PLoS Pathog. 2024 Sep 6;20(9):e1012509. doi: 10.1371/journal.ppat.1012509. eCollection 2024 Sep. PLoS Pathog. 2024. PMID: 39241103 Free PMC article.
-
The dependence of viral RNA replication on co-opted host factors.Nat Rev Microbiol. 2011 Dec 19;10(2):137-49. doi: 10.1038/nrmicro2692. Nat Rev Microbiol. 2011. PMID: 22183253 Free PMC article. Review.
-
Usutu virus: an emerging flavivirus in Europe.Viruses. 2015 Jan 19;7(1):219-38. doi: 10.3390/v7010219. Viruses. 2015. PMID: 25606971 Free PMC article. Review.
References
-
- Moss B, et al. Fields Virology. 2001. pp. 2849–2883.
Publication types
MeSH terms
LinkOut - more resources
Full Text Sources
Other Literature Sources