Notch signaling maintains bone marrow mesenchymal progenitors by suppressing osteoblast differentiation
- PMID: 18297083
- PMCID: PMC2740725
- DOI: 10.1038/nm1716
Notch signaling maintains bone marrow mesenchymal progenitors by suppressing osteoblast differentiation
Abstract
Postnatal bone marrow houses mesenchymal progenitor cells that are osteoblast precursors. These cells have established therapeutic potential, but they are difficult to maintain and expand in vitro, presumably because little is known about the mechanisms controlling their fate decisions. To investigate the potential role of Notch signaling in osteoblastogenesis, we used conditional alleles to genetically remove components of the Notch signaling system during skeletal development. We found that disruption of Notch signaling in the limb skeletogenic mesenchyme markedly increased trabecular bone mass in adolescent mice. Notably, mesenchymal progenitors were undetectable in the bone marrow of mice with high bone mass. As a result, these mice developed severe osteopenia as they aged. Moreover, Notch signaling seemed to inhibit osteoblast differentiation through Hes or Hey proteins, which diminished Runx2 transcriptional activity via physical interaction. These results support a model wherein Notch signaling in bone marrow normally acts to maintain a pool of mesenchymal progenitors by suppressing osteoblast differentiation. Thus, mesenchymal progenitors may be expanded in vitro by activating the Notch pathway, whereas bone formation in vivo may be enhanced by transiently suppressing this pathway.
Figures
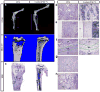
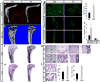
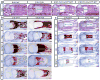
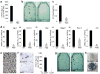
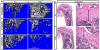
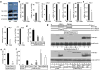
Similar articles
-
Physiological notch signaling maintains bone homeostasis via RBPjk and Hey upstream of NFATc1.PLoS Genet. 2012;8(3):e1002577. doi: 10.1371/journal.pgen.1002577. Epub 2012 Mar 22. PLoS Genet. 2012. PMID: 22457635 Free PMC article.
-
Targeting intercellular signals for bone regeneration from bone marrow mesenchymal progenitors.Cell Cycle. 2008 Jul 15;7(14):2106-11. doi: 10.4161/cc.7.14.6257. Epub 2008 May 8. Cell Cycle. 2008. PMID: 18635951
-
Jagged1 expression by osteoblast-lineage cells regulates trabecular bone mass and periosteal expansion in mice.Bone. 2016 Oct;91:64-74. doi: 10.1016/j.bone.2016.07.006. Epub 2016 Jul 12. Bone. 2016. PMID: 27416809 Free PMC article.
-
Alteration of Notch signaling in skeletal development and disease.Ann N Y Acad Sci. 2010 Mar;1192:257-68. doi: 10.1111/j.1749-6632.2009.05307.x. Ann N Y Acad Sci. 2010. PMID: 20392245 Free PMC article. Review.
-
Contextual Regulation of Skeletal Physiology by Notch Signaling.Curr Osteoporos Rep. 2019 Aug;17(4):217-225. doi: 10.1007/s11914-019-00516-y. Curr Osteoporos Rep. 2019. PMID: 31069622 Review.
Cited by
-
Hes1: a key role in stemness, metastasis and multidrug resistance.Cancer Biol Ther. 2015;16(3):353-9. doi: 10.1080/15384047.2015.1016662. Cancer Biol Ther. 2015. PMID: 25781910 Free PMC article. Review.
-
Nuclear factor of activated T-cells (NFAT)C2 inhibits Notch receptor signaling in osteoblasts.J Biol Chem. 2013 Jan 4;288(1):624-32. doi: 10.1074/jbc.M112.340455. Epub 2012 Nov 19. J Biol Chem. 2013. PMID: 23166323 Free PMC article.
-
Physical Activity and Post-Transcriptional Regulation of Aging Decay: Modulation of Pathways in Postmenopausal Osteoporosis.Medicina (Kaunas). 2022 Jun 6;58(6):767. doi: 10.3390/medicina58060767. Medicina (Kaunas). 2022. PMID: 35744030 Free PMC article. Review.
-
One-bone forearm procedure for Hajdu-Cheney syndrome: a case report.Hand (N Y). 2013 Dec;8(4):479-82. doi: 10.1007/s11552-013-9542-5. Hand (N Y). 2013. PMID: 24426971 Free PMC article.
-
Transcriptional responses of skeletal stem/progenitor cells to hindlimb unloading and recovery correlate with localized but not systemic multi-systems impacts.NPJ Microgravity. 2021 Nov 26;7(1):49. doi: 10.1038/s41526-021-00178-0. NPJ Microgravity. 2021. PMID: 34836964 Free PMC article.
References
-
- Bianco P, Riminucci M, Gronthos S, Robey PG. Bone marrow stromal stem cells: nature, biology, and potential applications. Stem Cells. 2001;19:180–92. - PubMed
-
- Artavanis-Tsakonas S, Rand MD, Lake RJ. Notch signaling: cell fate control and signal integration in development. Science. 1999;284:770–6. - PubMed
-
- Chiba S. Notch signaling in stem cell systems. Stem Cells. 2006;24:2437–47. - PubMed
-
- Schroeter EH, Kisslinger JA, Kopan R. Notch-1 signalling requires ligand-induced proteolytic release of intracellular domain. Nature. 1998;393:382–6. - PubMed
-
- Honjo T. The shortest path from the surface to the nucleus: RBP-J kappa/Su(H) transcription factor. Genes Cells. 1996;1:1–9. - PubMed
Publication types
MeSH terms
Substances
Grants and funding
- R01 HD044056-05/HD/NICHD NIH HHS/United States
- AR046852/AR/NIAMS NIH HHS/United States
- R01 AR046852/AR/NIAMS NIH HHS/United States
- R01 HD044056/HD/NICHD NIH HHS/United States
- HD044056/HD/NICHD NIH HHS/United States
- DK065789/DK/NIDDK NIH HHS/United States
- DK11794/DK/NIDDK NIH HHS/United States
- T32 AR007033/AR/NIAMS NIH HHS/United States
- R01 AR046523/AR/NIAMS NIH HHS/United States
- 5T32AR07033/AR/NIAMS NIH HHS/United States
- R37 AR046523/AR/NIAMS NIH HHS/United States
- R01 DK065789/DK/NIDDK NIH HHS/United States
- R01 DK065789-05/DK/NIDDK NIH HHS/United States
- AR046523/AR/NIAMS NIH HHS/United States
LinkOut - more resources
Full Text Sources
Other Literature Sources
Molecular Biology Databases
Research Materials