A Drosophila model for LRRK2-linked parkinsonism
- PMID: 18258746
- PMCID: PMC2268198
- DOI: 10.1073/pnas.0708452105
A Drosophila model for LRRK2-linked parkinsonism
Abstract
Mutations in the leucine-rich repeat kinase (LRRK2) gene cause late-onset autosomal dominant Parkinson's disease (PD) with pleiomorphic pathology. Previously, we and others found that expression of mutant LRRK2 causes neuronal degeneration in cell culture. Here we used the GAL4/UAS system to generate transgenic Drosophila expressing either wild-type human LRRK2 or LRRK2-G2019S, the most common mutation associated with PD. Expression of either wild-type human LRRK2 or LRRK2-G2019S in the photoreceptor cells caused retinal degeneration. Expression of LRRK2 or LRRK2-G2019S in neurons produced adult-onset selective loss of dopaminergic neurons, locomotor dysfunction, and early mortality. Expression of mutant G2019S-LRRK2 caused a more severe parkinsonism-like phenotype than expression of equivalent levels of wild-type LRRK2. Treatment with l-DOPA improved mutant LRRK2-induced locomotor impairment but did not prevent the loss of tyrosine hydroxylase-positive neurons. To our knowledge, this is the first in vivo"gain-of-function" model which recapitulates several key features of LRRK2-linked human parkinsonism. These flies may provide a useful model for studying LRRK2-linked pathogenesis and for future therapeutic screens for PD intervention.
Conflict of interest statement
The authors declare no conflict of interest.
Figures
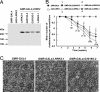
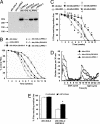
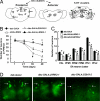
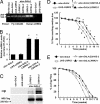
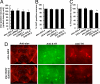
Similar articles
-
Lovastatin protects neurite degeneration in LRRK2-G2019S parkinsonism through activating the Akt/Nrf pathway and inhibiting GSK3β activity.Hum Mol Genet. 2016 May 15;25(10):1965-1978. doi: 10.1093/hmg/ddw068. Epub 2016 Feb 29. Hum Mol Genet. 2016. PMID: 26931464
-
The Drosophila hep pathway mediates Lrrk2-induced neurodegeneration.Biochem Cell Biol. 2018 Aug;96(4):441-449. doi: 10.1139/bcb-2017-0262. Epub 2017 Dec 21. Biochem Cell Biol. 2018. PMID: 29268033 Free PMC article.
-
LRRK2-mediated neurodegeneration and dysfunction of dopaminergic neurons in a Caenorhabditis elegans model of Parkinson's disease.Neurobiol Dis. 2010 Oct;40(1):73-81. doi: 10.1016/j.nbd.2010.04.002. Epub 2010 Apr 9. Neurobiol Dis. 2010. PMID: 20382224 Free PMC article.
-
Co-occurrence of sporadic parkinsonism and late-onset Alzheimer's disease in a Brazilian male with the LRRK2 p.G2019S mutation.Genet Test. 2008 Dec;12(4):471-3. doi: 10.1089/gte.2008.0042. Genet Test. 2008. PMID: 19072560 Review.
-
The synaptic function of LRRK2.Biochem Soc Trans. 2012 Oct;40(5):1047-51. doi: 10.1042/BST20120113. Biochem Soc Trans. 2012. PMID: 22988863 Review.
Cited by
-
LRRK2 Attenuates Antioxidant Response in Familial Parkinson's Disease Derived Neural Stem Cells.Cells. 2023 Oct 31;12(21):2550. doi: 10.3390/cells12212550. Cells. 2023. PMID: 37947628 Free PMC article.
-
Mitochondrial dysfunction in Parkinson's disease: molecular mechanisms and pathophysiological consequences.EMBO J. 2012 Jun 26;31(14):3038-62. doi: 10.1038/emboj.2012.170. EMBO J. 2012. PMID: 22735187 Free PMC article. Review.
-
Human Induced Pluripotent Stem Cell Phenotyping and Preclinical Modeling of Familial Parkinson's Disease.Genes (Basel). 2022 Oct 25;13(11):1937. doi: 10.3390/genes13111937. Genes (Basel). 2022. PMID: 36360174 Free PMC article. Review.
-
Parkin Knockdown Modulates Dopamine Release in the Central Complex, but Not the Mushroom Body Heel, of Aging Drosophila.ACS Chem Neurosci. 2023 Jan 18;14(2):198-208. doi: 10.1021/acschemneuro.2c00277. Epub 2022 Dec 28. ACS Chem Neurosci. 2023. PMID: 36576890 Free PMC article.
-
Gene-environment interactions: key to unraveling the mystery of Parkinson's disease.Prog Neurobiol. 2011 Jun;94(1):1-19. doi: 10.1016/j.pneurobio.2011.03.005. Epub 2011 Mar 23. Prog Neurobiol. 2011. PMID: 21439347 Free PMC article. Review.
References
-
- Paisan-Ruiz C, Jain S, Evans EW, Gilks WP, Simon J, van der Brug M, Lopez de Munain A, Aparicio S, Gil AM, Khan N, et al. Neuron. 2004;44:595–600. - PubMed
-
- Zimprich A, Biskup S, Leitner P, Lichtner P, Farrer M, Lincoln S, Kachergus J, Hulihan M, Uitti RJ, Calne DB, et al. Neuron. 2004;44:601–607. - PubMed
-
- Ross OA, Toft M, Whittle AJ, Johnson JL, Papapetropoulos S, Mash DC, Litvan I, Gordon MF, Wszolek ZK, Farrer MJ, et al. Ann Neurol. 2006;59:388–393. - PubMed
-
- Rajput A, Dickson DW, Robinson CA, Ross OA, Dachsel JC, Lincoln SJ, Cobb SA, Rajput ML, Farrer MJ. Neurology. 2006;67:1506–1508. - PubMed
-
- MacLeod D, Dowman J, Hammond R, Leete T, Inoue K, Abeliovich A. Neuron. 2006;52:587–593. - PubMed
Publication types
MeSH terms
Substances
Grants and funding
LinkOut - more resources
Full Text Sources
Other Literature Sources
Molecular Biology Databases
Miscellaneous