Glial dysfunction in parkin null mice: effects of aging
- PMID: 18199761
- PMCID: PMC6670347
- DOI: 10.1523/JNEUROSCI.4609-07.2008
Glial dysfunction in parkin null mice: effects of aging
Abstract
Parkin mutations in humans produce parkinsonism whose pathogenesis is related to impaired protein degradation, increased free radicals, and abnormal neurotransmitter release. The role of glia in parkin deficiency is little known. We cultured midbrain glia from wild-type (WT) and parkin knock-out (PK-KO) mice. After 18-20 d in vitro, PK-KO glial cultures had less astrocytes, more microglia, reduced proliferation, and increased proapoptotic protein expression. PK-KO glia had greater levels of intracellular glutathione (GSH), increased mRNA expression of the GSH-synthesizing enzyme gamma-glutamylcysteine synthetase, and greater glutathione S-transferase and lower glutathione peroxidase activities than WT. The reverse happened in glia cultured in serum-free defined medium (EF12) or in old cultures. PK-KO glia was more susceptible than WT to transference to EF12 or neurotoxins (1-methyl-4-phenylpyridinium, blockers of GSH synthesis or catalase, inhibitors of extracellular signal-regulated kinase 1/2 and phosphatidylinositol 3 kinases), aging of the culture, or combination of these insults. PK-KO glia was less susceptible than WT to Fe2+ plus H2O2 and less responsive to protection by deferoxamine. Old WT glia increased the expression of heat shock protein 70, but PK-KO did not. Glia conditioned medium (GCM) from PK-KO was less neuroprotective and had lower levels of GSH than WT. GCM from WT increased the levels of dopamine markers in midbrain neuronal cultures transferred to EF12 more efficiently than GCM from PK-KO, and the difference was corrected by supplementation with GSH. PK-KO-GCM was a less powerful suppressor of apoptosis and microglia in neuronal cultures. Our data prove that abnormal glial function is critical in parkin mutations, and its role increases with aging.
Figures
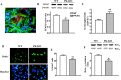
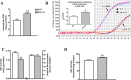
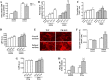
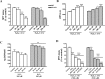
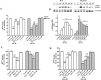
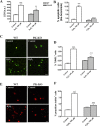
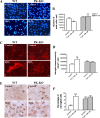
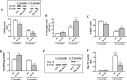
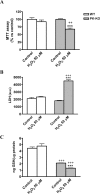
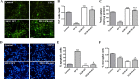
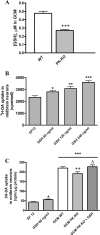
Similar articles
-
Parkin deficiency increases the resistance of midbrain neurons and glia to mild proteasome inhibition: the role of autophagy and glutathione homeostasis.J Neurochem. 2009 Sep;110(5):1523-37. doi: 10.1111/j.1471-4159.2009.06248.x. Epub 2009 Jun 22. J Neurochem. 2009. PMID: 19549073
-
Effects of cinnarizine, a calcium antagonist that produces human parkinsonism, in parkin knock out mice.Neuropharmacology. 2005 Aug;49(2):208-19. doi: 10.1016/j.neuropharm.2005.03.003. Neuropharmacology. 2005. PMID: 15993444
-
Differential effects of l-DOPA on monoamine metabolism, cell survival and glutathione production in midbrain neuronal-enriched cultures from parkin knockout and wild-type mice.J Neurochem. 2005 Aug;94(4):1005-14. doi: 10.1111/j.1471-4159.2005.03249.x. Epub 2005 Jul 5. J Neurochem. 2005. PMID: 16000163
-
Neurons, glia, and plasticity in normal brain aging.Neurobiol Aging. 2003 May-Jun;24 Suppl 1:S123-7; discussion S131. doi: 10.1016/s0197-4580(03)00051-4. Neurobiol Aging. 2003. PMID: 12829120 Review.
-
Role of glial cells in the generation of sex differences in neurodegenerative diseases and brain aging.Mech Ageing Dev. 2021 Jun;196:111473. doi: 10.1016/j.mad.2021.111473. Epub 2021 Mar 22. Mech Ageing Dev. 2021. PMID: 33766745 Review.
Cited by
-
Role of Astrogliosis in the Pathogenesis of Parkinson's Disease: Insights into Astrocytic Nrf2 Pathway as a Potential Therapeutic Target.CNS Neurol Disord Drug Targets. 2024;23(8):1015-1029. doi: 10.2174/0118715273270473231002104610. CNS Neurol Disord Drug Targets. 2024. PMID: 37817521 Review.
-
Astrocytes as targets for drug discovery.Drug Discov Today. 2018 Mar;23(3):673-680. doi: 10.1016/j.drudis.2018.01.011. Epub 2018 Jan 6. Drug Discov Today. 2018. PMID: 29317338 Free PMC article. Review.
-
The Role of Astrocyte Dysfunction in Parkinson's Disease Pathogenesis.Trends Neurosci. 2017 Jun;40(6):358-370. doi: 10.1016/j.tins.2017.04.001. Epub 2017 May 17. Trends Neurosci. 2017. PMID: 28527591 Free PMC article. Review.
-
Astrocytes in a dish: Using pluripotent stem cells to model neurodegenerative and neurodevelopmental disorders.Brain Pathol. 2017 Jul;27(4):530-544. doi: 10.1111/bpa.12522. Brain Pathol. 2017. PMID: 28585380 Free PMC article.
-
Impaired inflammatory responses in murine Lrrk2-knockdown brain microglia.PLoS One. 2012;7(4):e34693. doi: 10.1371/journal.pone.0034693. Epub 2012 Apr 9. PLoS One. 2012. PMID: 22496842 Free PMC article.
References
-
- Abbas N, Lucking CB, Ricard S, Durr A, Bonifati V, De Michele G, Bouley S, Vaughan JR, Gasser T, Marconi R, Broussolle E, Brefel-Courbon C, Harhangi BS, Oostra BA, Fabrizio E, Bohme GA, Pradier L, Wood NW, Filla A, Meco G, Denefle P, Agid Y, Brice A. A wide variety of mutations in the parkin gene are responsible for autosomal recessive parkinsonism in Europe. French Parkinson's Disease Genetics Study Group and the European Consortium on Genetic Susceptibility in Parkinson's Disease. Hum Mol Genet. 1999;8:567–574. - PubMed
-
- Andringa G, Bol JG, Wang X, Boekel A, Bennett MC, Chase TN, Drukarch B. Changed distribution pattern of the constitutive rather than the inducible HSP70 chaperone in neuromelanin-containing neurones of the Parkinsonian midbrain. Neuropathol Appl Neurobiol. 2006;32:157–169. - PubMed
-
- Arenas E. Stem cells in the treatment of Parkinson's disease. Brain Res Bull. 2002;57:795–808. - PubMed
-
- Ashwell K. The distribution of microglia and cell death in the fetal rat forebrain. Brain Res Dev Brain Res. 1991;58:1–12. - PubMed
-
- Barker JE, Heales SJ, Cassidy A, Bolanos JP, Land JM, Clark JB. Depletion of brain glutathione results in a decrease of glutathione reductase activity; an enzyme susceptible to oxidative damage. Brain Res. 1996;716:118–122. - PubMed
Publication types
MeSH terms
Substances
LinkOut - more resources
Full Text Sources
Other Literature Sources
Medical
Research Materials