Roles of Greatwall kinase in the regulation of cdc25 phosphatase
- PMID: 18199678
- PMCID: PMC2291418
- DOI: 10.1091/mbc.e07-11-1099
Roles of Greatwall kinase in the regulation of cdc25 phosphatase
Abstract
We previously reported that immunodepletion of Greatwall kinase prevents Xenopus egg extracts from entering or maintaining M phase due to the accumulation of inhibitory phosphorylations on Thr14 and Tyr15 of Cdc2. M phase-promoting factor (MPF) in turn activates Greatwall, implying that Greatwall participates in an MPF autoregulatory loop. We show here that activated Greatwall both accelerates the mitotic G2/M transition in cycling egg extracts and induces meiotic maturation in G2-arrested Xenopus oocytes in the absence of progesterone. Activated Greatwall can induce phosphorylations of Cdc25 in the absence of the activity of Cdc2, Plx1 (Xenopus Polo-like kinase) or mitogen-activated protein kinase, or in the presence of an activator of protein kinase A that normally blocks mitotic entry. The effects of active Greatwall mimic in many respects those associated with addition of the phosphatase inhibitor okadaic acid (OA); moreover, OA allows cycling extracts to enter M phase in the absence of Greatwall. Taken together, these findings support a model in which Greatwall negatively regulates a crucial phosphatase that inhibits Cdc25 activation and M phase induction.
Figures
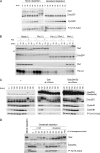
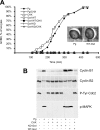
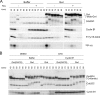
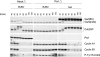
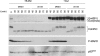
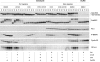
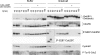
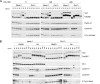
Similar articles
-
Greatwall kinase participates in the Cdc2 autoregulatory loop in Xenopus egg extracts.Mol Cell. 2006 Apr 7;22(1):83-91. doi: 10.1016/j.molcel.2006.02.022. Mol Cell. 2006. PMID: 16600872
-
Constant regulation of both the MPF amplification loop and the Greatwall-PP2A pathway is required for metaphase II arrest and correct entry into the first embryonic cell cycle.J Cell Sci. 2010 Jul 1;123(Pt 13):2281-91. doi: 10.1242/jcs.064527. J Cell Sci. 2010. PMID: 20554897
-
Polo-like kinase confers MPF autoamplification competence to growing Xenopus oocytes.Development. 2004 Apr;131(7):1543-52. doi: 10.1242/dev.01050. Epub 2004 Feb 25. Development. 2004. PMID: 14985258
-
Phosphatases driving mitosis: pushing the gas and lifting the brakes.Prog Mol Biol Transl Sci. 2012;106:327-41. doi: 10.1016/B978-0-12-396456-4.00008-0. Prog Mol Biol Transl Sci. 2012. PMID: 22340723 Review.
-
Greatwall kinase, ARPP-19 and protein phosphatase 2A: shifting the mitosis paradigm.Results Probl Cell Differ. 2011;53:219-34. doi: 10.1007/978-3-642-19065-0_11. Results Probl Cell Differ. 2011. PMID: 21630148 Review.
Cited by
-
The M phase kinase Greatwall (Gwl) promotes inactivation of PP2A/B55delta, a phosphatase directed against CDK phosphosites.Mol Biol Cell. 2009 Nov;20(22):4777-89. doi: 10.1091/mbc.e09-07-0643. Epub 2009 Sep 30. Mol Biol Cell. 2009. PMID: 19793917 Free PMC article.
-
Canonical and Alternative Pathways in Cyclin-Dependent Kinase 1/Cyclin B Inactivation upon M-Phase Exit in Xenopus laevis Cell-Free Extracts.Enzyme Res. 2011;2011:523420. doi: 10.4061/2011/523420. Epub 2011 Jun 22. Enzyme Res. 2011. PMID: 21755042 Free PMC article.
-
Two Interlinked Bistable Switches Govern Mitotic Control in Mammalian Cells.Curr Biol. 2018 Dec 3;28(23):3824-3832.e6. doi: 10.1016/j.cub.2018.09.059. Epub 2018 Nov 15. Curr Biol. 2018. PMID: 30449668 Free PMC article.
-
Genome wide decrease of DNA replication eye density at the midblastula transition of Xenopus laevis.Cell Cycle. 2019 Jul;18(13):1458-1472. doi: 10.1080/15384101.2019.1618641. Epub 2019 May 26. Cell Cycle. 2019. PMID: 31130065 Free PMC article.
-
Role for regulated phosphatase activity in generating mitotic oscillations in Xenopus cell-free extracts.Proc Natl Acad Sci U S A. 2013 Dec 17;110(51):20539-44. doi: 10.1073/pnas.1318065110. Epub 2013 Dec 2. Proc Natl Acad Sci U S A. 2013. PMID: 24297885 Free PMC article.
References
-
- Abrieu A., Brassac T., Galas S., Fisher D., Labbe J., Doree M. The Polo-like kinase Plx1 is a component of the MPF amplification loop at the G2/M-phase transition of the cell cycle in Xenopus eggs. J. Cell Sci. 1998;111:1751–1757. - PubMed
-
- Barr F. A., Sillje H.H.W., Nigg E. A. Polo-like kinases and the orchestration of cell division. Nat. Rev. Mol. Cell Biol. 2004;5:429–441. - PubMed
-
- Chun J., Chau A. S., Maingat F. G., Edmonds S. D., Ostergaard H. L., Shibuya E. K. Phosphorylation of Cdc25C by pp90Rsk contributes to a G2 cell cycle arrest in Xenopus cycling egg extracts. Cell Cycle. 2005;4:148–154. - PubMed
Publication types
MeSH terms
Substances
Grants and funding
LinkOut - more resources
Full Text Sources
Other Literature Sources
Research Materials
Miscellaneous