Suppression of neurodegeneration and increased neurotransmission caused by expanded full-length huntingtin accumulating in the cytoplasm
- PMID: 18184562
- PMCID: PMC2277511
- DOI: 10.1016/j.neuron.2007.11.025
Suppression of neurodegeneration and increased neurotransmission caused by expanded full-length huntingtin accumulating in the cytoplasm
Abstract
Huntington's disease (HD) is a dominantly inherited neurodegenerative disorder caused by expansion of a translated CAG repeat in the N terminus of the huntingtin (htt) protein. Here we describe the generation and characterization of a full-length HD Drosophila model to reveal a previously unknown disease mechanism that occurs early in the course of pathogenesis, before expanded htt is imported into the nucleus in detectable amounts. We find that expanded full-length htt (128Qhtt(FL)) leads to behavioral, neurodegenerative, and electrophysiological phenotypes. These phenotypes are caused by a Ca2+-dependent increase in neurotransmitter release efficiency in 128Qhtt(FL) animals. Partial loss of function in synaptic transmission (syntaxin, Snap, Rop) and voltage-gated Ca2+ channel genes suppresses both the electrophysiological and the neurodegenerative phenotypes. Thus, our data indicate that increased neurotransmission is at the root of neuronal degeneration caused by expanded full-length htt during early stages of pathogenesis.
Figures
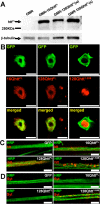
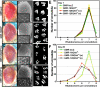
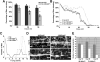
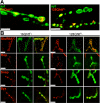
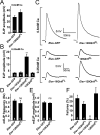
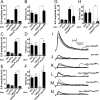
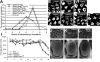
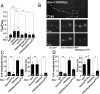
Similar articles
-
Inducing huntingtin inclusion formation in primary neuronal cell culture and in vivo by high-capacity adenoviral vectors expressing truncated and full-length huntingtin with polyglutamine expansion.J Gene Med. 2008 Mar;10(3):269-79. doi: 10.1002/jgm.1150. J Gene Med. 2008. PMID: 18067195
-
Cytoplasmic aggregates trap polyglutamine-containing proteins and block axonal transport in a Drosophila model of Huntington's disease.Proc Natl Acad Sci U S A. 2004 Mar 2;101(9):3224-9. doi: 10.1073/pnas.0400243101. Epub 2004 Feb 20. Proc Natl Acad Sci U S A. 2004. PMID: 14978262 Free PMC article.
-
Early Neurodegeneration in R6/2 Mice Carrying the Huntington's Disease Mutation with a Super-Expanded CAG Repeat, Despite Normal Lifespan.J Huntingtons Dis. 2018;7(1):61-76. doi: 10.3233/JHD-170265. J Huntingtons Dis. 2018. PMID: 29480204
-
Selective degeneration in YAC mouse models of Huntington disease.Brain Res Bull. 2007 Apr 30;72(2-3):124-31. doi: 10.1016/j.brainresbull.2006.10.018. Epub 2006 Nov 16. Brain Res Bull. 2007. PMID: 17352936 Review.
-
Advances in huntington disease drug discovery: novel approaches to model disease phenotypes.J Biomol Screen. 2014 Feb;19(2):191-204. doi: 10.1177/1087057113510320. Epub 2013 Nov 6. J Biomol Screen. 2014. PMID: 24196395 Review.
Cited by
-
Huntingtin proteolysis releases non-polyQ fragments that cause toxicity through dynamin 1 dysregulation.EMBO J. 2015 Sep 2;34(17):2255-71. doi: 10.15252/embj.201490808. Epub 2015 Jul 12. EMBO J. 2015. PMID: 26165689 Free PMC article.
-
Attenuating the DNA damage response to double-strand breaks restores function in models of CNS neurodegeneration.Brain Commun. 2019 Jul 2;1(1):fcz005. doi: 10.1093/braincomms/fcz005. eCollection 2019. Brain Commun. 2019. PMID: 32954257 Free PMC article.
-
Targeting microglia L-type voltage-dependent calcium channels for the treatment of central nervous system disorders.J Neurosci Res. 2021 Jan;99(1):141-162. doi: 10.1002/jnr.24585. Epub 2020 Jan 29. J Neurosci Res. 2021. PMID: 31997405 Free PMC article. Review.
-
Pathogenic Huntington Alters BMP Signaling and Synaptic Growth through Local Disruptions of Endosomal Compartments.J Neurosci. 2017 Mar 22;37(12):3425-3439. doi: 10.1523/JNEUROSCI.2752-16.2017. Epub 2017 Feb 24. J Neurosci. 2017. PMID: 28235896 Free PMC article.
-
Fructose-2,6-bisphosphate restores DNA repair activity of PNKP and ameliorates neurodegenerative symptoms in Huntington's disease.Proc Natl Acad Sci U S A. 2024 Sep 24;121(39):e2406308121. doi: 10.1073/pnas.2406308121. Epub 2024 Sep 19. Proc Natl Acad Sci U S A. 2024. PMID: 39298485
References
-
- The Huntington's Disease Collaborative Research Group A novel gene containing a trinucleotide repeat that is expanded and unstable on Huntington's disease chromosomes. Cell. 1993;72:971–983. - PubMed
-
- Auluck PK, Chan HY, Trojanowski JQ, Lee VM, Bonini NM. Chaperone suppression of alpha-synuclein toxicity in a Drosophila model for Parkinson's disease. Science. 2002;295:865–868. - PubMed
-
- Bezprozvanny I, Hayden MR. Deranged neuronal calcium signaling and Huntington disease. Biochem Biophys Res Commun. 2004;322:1310–1317. - PubMed
-
- Bilen J, Bonini NM. Drosophila as a model for human neurodegenerative disease. Annu Rev Genet. 2005;39:153–171. - PubMed
-
- Brand AH, Perrimon N. Targeted gene expression as a means of altering cell fates and generating dominant phenotypes. Development. 1993;118:401–415. - PubMed
Publication types
MeSH terms
Substances
Grants and funding
LinkOut - more resources
Full Text Sources
Other Literature Sources
Molecular Biology Databases
Miscellaneous