The Legionella pneumophila IcmSW complex interacts with multiple Dot/Icm effectors to facilitate type IV translocation
- PMID: 18069892
- PMCID: PMC2134951
- DOI: 10.1371/journal.ppat.0030188
The Legionella pneumophila IcmSW complex interacts with multiple Dot/Icm effectors to facilitate type IV translocation
Abstract
Many gram-negative pathogens use a type IV secretion system (T4SS) to deliver effector proteins into eukaryotic host cells. The fidelity of protein translocation depends on the efficient recognition of effector proteins by the T4SS. Legionella pneumophila delivers a large number of effector proteins into eukaryotic cells using the Dot/Icm T4SS. How the Dot/Icm system is able to recognize and control the delivery of effectors is poorly understood. Recent studies suggest that the IcmS and IcmW proteins interact to form a stable complex that facilitates translocation of effector proteins by the Dot/Icm system by an unknown mechanism. Here we demonstrate that the IcmSW complex is necessary for the productive translocation of multiple Dot/Icm effector proteins. Effector proteins that were able to bind IcmSW in vitro required icmS and icmW for efficient translocation into eukaryotic cells during L. pneumophila infection. We identified regions in the effector protein SidG involved in icmSW-dependent translocation. Although the full-length SidG protein was translocated by an icmSW-dependent mechanism, deletion of amino terminal regions in the SidG protein resulted in icmSW-independent translocation, indicating that the IcmSW complex is not contributing directly to recognition of effector proteins by the Dot/Icm system. Biochemical and genetic studies showed that the IcmSW complex interacts with a central region of the SidG protein. The IcmSW interaction resulted in a conformational change in the SidG protein as determined by differences in protease sensitivity in vitro. These data suggest that IcmSW binding to effectors could enhance effector protein delivery by mediating a conformational change that facilitates T4SS recognition of a translocation domain located in the carboxyl region of the effector protein.
Conflict of interest statement
Figures
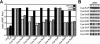
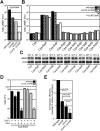
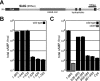
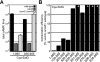
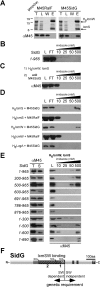
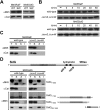
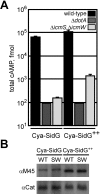
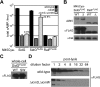
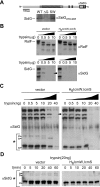
Similar articles
-
A Legionella pneumophila effector protein encoded in a region of genomic plasticity binds to Dot/Icm-modified vacuoles.PLoS Pathog. 2009 Jan;5(1):e1000278. doi: 10.1371/journal.ppat.1000278. Epub 2009 Jan 23. PLoS Pathog. 2009. PMID: 19165328 Free PMC article.
-
The Legionella IcmSW complex directly interacts with DotL to mediate translocation of adaptor-dependent substrates.PLoS Pathog. 2012 Sep;8(9):e1002910. doi: 10.1371/journal.ppat.1002910. Epub 2012 Sep 13. PLoS Pathog. 2012. PMID: 23028312 Free PMC article.
-
Dependency of Coxiella burnetii Type 4B Secretion on the Chaperone IcmS.J Bacteriol. 2019 Nov 5;201(23):e00431-19. doi: 10.1128/JB.00431-19. Print 2019 Dec 1. J Bacteriol. 2019. PMID: 31501284 Free PMC article.
-
Legionella pneumophila, armed to the hilt: justifying the largest arsenal of effectors in the bacterial world.Curr Opin Microbiol. 2016 Feb;29:74-80. doi: 10.1016/j.mib.2015.11.002. Epub 2015 Dec 19. Curr Opin Microbiol. 2016. PMID: 26709975 Review.
-
Effector translocation by the Legionella Dot/Icm type IV secretion system.Curr Top Microbiol Immunol. 2013;376:103-15. doi: 10.1007/82_2013_345. Curr Top Microbiol Immunol. 2013. PMID: 23918176 Review.
Cited by
-
VirJ Is a Brucella Virulence Factor Involved in the Secretion of Type IV Secreted Substrates.J Biol Chem. 2016 Jun 3;291(23):12383-93. doi: 10.1074/jbc.M116.730994. Epub 2016 Apr 8. J Biol Chem. 2016. PMID: 27059960 Free PMC article.
-
Recent advances in structural studies of the Legionella pneumophila Dot/Icm type IV secretion system.Microbiol Immunol. 2022 Feb;66(2):67-74. doi: 10.1111/1348-0421.12951. Epub 2022 Jan 17. Microbiol Immunol. 2022. PMID: 34807482 Free PMC article. Review.
-
A Dot/Icm-translocated ankyrin protein of Legionella pneumophila is required for intracellular proliferation within human macrophages and protozoa.Mol Microbiol. 2008 Nov;70(4):908-23. doi: 10.1111/j.1365-2958.2008.06453.x. Epub 2008 Sep 22. Mol Microbiol. 2008. PMID: 18811729 Free PMC article.
-
A Legionella pneumophila effector protein encoded in a region of genomic plasticity binds to Dot/Icm-modified vacuoles.PLoS Pathog. 2009 Jan;5(1):e1000278. doi: 10.1371/journal.ppat.1000278. Epub 2009 Jan 23. PLoS Pathog. 2009. PMID: 19165328 Free PMC article.
-
Life Stage-specific Proteomes of Legionella pneumophila Reveal a Highly Differential Abundance of Virulence-associated Dot/Icm effectors.Mol Cell Proteomics. 2016 Jan;15(1):177-200. doi: 10.1074/mcp.M115.053579. Epub 2015 Nov 6. Mol Cell Proteomics. 2016. PMID: 26545400 Free PMC article.
References
-
- Berger KH, Isberg RR. Two distinct defects in intracellular growth complemented by a single genetic locus in Legionella pneumophila . Mol Microbiol. 1993;7:7–19. - PubMed
-
- Vogel JP, Andrews HL, Wong SK, Isberg RR. Conjugative transfer by the virulence system of Legionella pneumophila . Science. 1998;279:873–876. - PubMed
-
- Roy CR, Berger KH, Isberg RR. Legionella pneumophila DotA protein is required for early phagosome trafficking decisions that occur within minutes of bacterial uptake. Mol Microbiol. 1998;28:663–674. - PubMed
Publication types
MeSH terms
Substances
Grants and funding
LinkOut - more resources
Full Text Sources
Medical