Measurement of bond vector orientations in invisible excited states of proteins
- PMID: 18006656
- PMCID: PMC2141801
- DOI: 10.1073/pnas.0708296104
Measurement of bond vector orientations in invisible excited states of proteins
Abstract
The focus of structural biology is on studies of the highly populated, ground states of biological molecules; states that are only sparsely and transiently populated are more difficult to probe because they are invisible to most structural methods. Yet, such states can play critical roles in biochemical processes such as ligand binding, enzyme catalysis, and protein folding. A description of these states in terms of structure and dynamics is, therefore, of great importance. Here, we present a method, based on relaxation dispersion NMR spectroscopy of weakly aligned molecules in a magnetic field, that can provide such a description by direct measurement of backbone amide bond vector orientations in transient, low populated states that are not observable directly. Such information, obtained through the measurement of residual dipolar couplings, has until now been restricted to proteins that produce observable spectra. The methodology is applied and validated in a study of the binding of a target peptide to an SH3 domain from the yeast protein Abp1p and subsequently used in an application to protein folding of a mutational variant of the Fyn SH3 domain where (1)H-(15)N dipolar couplings of the invisible unfolded state of the domain are obtained. The approach, which can be used to obtain orientational restraints at other sites in proteins as well, promises to significantly extend the available information necessary for providing a site-specific characterization of structural properties of transient, low populated states that have to this point remained recalcitrant to detailed analysis.
Conflict of interest statement
The authors declare no conflict of interest.
Figures
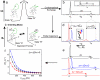
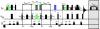
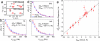
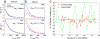
Similar articles
-
Depressing time: Waiting, melancholia, and the psychoanalytic practice of care.In: Kirtsoglou E, Simpson B, editors. The Time of Anthropology: Studies of Contemporary Chronopolitics. Abingdon: Routledge; 2020. Chapter 5. In: Kirtsoglou E, Simpson B, editors. The Time of Anthropology: Studies of Contemporary Chronopolitics. Abingdon: Routledge; 2020. Chapter 5. PMID: 36137063 Free Books & Documents. Review.
-
Qualitative evidence synthesis informing our understanding of people's perceptions and experiences of targeted digital communication.Cochrane Database Syst Rev. 2019 Oct 23;10(10):ED000141. doi: 10.1002/14651858.ED000141. Cochrane Database Syst Rev. 2019. PMID: 31643081 Free PMC article.
-
Enabling Systemic Identification and Functionality Profiling for Cdc42 Homeostatic Modulators.bioRxiv [Preprint]. 2024 Jan 8:2024.01.05.574351. doi: 10.1101/2024.01.05.574351. bioRxiv. 2024. Update in: Commun Chem. 2024 Nov 19;7(1):271. doi: 10.1038/s42004-024-01352-7. PMID: 38260445 Free PMC article. Updated. Preprint.
-
Comparison of Two Modern Survival Prediction Tools, SORG-MLA and METSSS, in Patients With Symptomatic Long-bone Metastases Who Underwent Local Treatment With Surgery Followed by Radiotherapy and With Radiotherapy Alone.Clin Orthop Relat Res. 2024 Dec 1;482(12):2193-2208. doi: 10.1097/CORR.0000000000003185. Epub 2024 Jul 23. Clin Orthop Relat Res. 2024. PMID: 39051924
-
Trends in Surgical and Nonsurgical Aesthetic Procedures: A 14-Year Analysis of the International Society of Aesthetic Plastic Surgery-ISAPS.Aesthetic Plast Surg. 2024 Oct;48(20):4217-4227. doi: 10.1007/s00266-024-04260-2. Epub 2024 Aug 5. Aesthetic Plast Surg. 2024. PMID: 39103642 Review.
Cited by
-
Structural dynamics of human deoxyuridine 5'-triphosphate nucleotidohydrolase (dUTPase).Sci Rep. 2024 Oct 30;14(1):26081. doi: 10.1038/s41598-024-76548-x. Sci Rep. 2024. PMID: 39477983 Free PMC article.
-
Is buffer a good proxy for a crowded cell-like environment? A comparative NMR study of calmodulin side-chain dynamics in buffer and E. coli lysate.PLoS One. 2012;7(10):e48226. doi: 10.1371/journal.pone.0048226. Epub 2012 Oct 30. PLoS One. 2012. PMID: 23118958 Free PMC article.
-
Isotope labeling methods for studies of excited protein states by relaxation dispersion NMR spectroscopy.Nat Protoc. 2009;4(11):1641-8. doi: 10.1038/nprot.2009.118. Epub 2009 Oct 22. Nat Protoc. 2009. PMID: 19876024
-
Probing excited states and activation energy for the integral membrane protein phospholamban by NMR CPMG relaxation dispersion experiments.Biochim Biophys Acta. 2010 Feb;1798(2):77-81. doi: 10.1016/j.bbamem.2009.09.009. Epub 2009 Sep 23. Biochim Biophys Acta. 2010. PMID: 19781521 Free PMC article.
-
Paramagnetic Chemical Probes for Studying Biological Macromolecules.Chem Rev. 2022 May 25;122(10):9571-9642. doi: 10.1021/acs.chemrev.1c00708. Epub 2022 Jan 27. Chem Rev. 2022. PMID: 35084831 Free PMC article. Review.
References
-
- Palmer AG, Williams J, McDermott A. J Phys Chem. 1996;100:13293–13310.
-
- Ishima R, Torchia DA. Nat Struct Biol. 2000;7:740–743. - PubMed
-
- Hahn EL. Phys Rev. 1950;80:580–594.
-
- Carr HY, Purcell EM. Phys Rev. 1954;4:630–638.
-
- Meiboom S, Gill D. Rev Sci Instrum. 1958;29:688–691.
MeSH terms
Substances
LinkOut - more resources
Full Text Sources
Molecular Biology Databases
Miscellaneous