Portal motor velocity and internal force resisting viral DNA packaging in bacteriophage phi29
- PMID: 17827233
- PMCID: PMC2134861
- DOI: 10.1529/biophysj.107.104612
Portal motor velocity and internal force resisting viral DNA packaging in bacteriophage phi29
Abstract
During the assembly of many viruses, a powerful molecular motor compacts the genome into a preassembled capsid. Here, we present measurements of viral DNA packaging in bacteriophage phi29 using an improved optical tweezers method that allows DNA translocation to be measured from initiation to completion. This method allowed us to study the previously uncharacterized early stages of packaging and facilitated more accurate measurement of the length of DNA packaged. We measured the motor velocity versus load at near-zero filling and developed a ramped DNA stretching technique that allowed us to measure the velocity versus capsid filling at near-zero load. These measurements reveal that the motor can generate significantly higher velocities and forces than detected previously. Toward the end of packaging, the internal force resisting DNA confinement rises steeply, consistent with the trend predicted by many theoretical models. However, the force rises to a higher magnitude, particularly during the early stages of packaging, than predicted by models that assume coaxial inverse spooling of the DNA. This finding suggests that the DNA is not arranged in that conformation during the early stages of packaging and indicates that internal force is available to drive complete genome ejection in vitro. The maximum force exceeds 100 pN, which is about one-half that predicted to rupture the capsid shell.
Figures
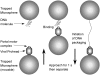
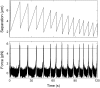
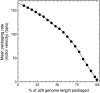
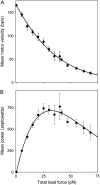
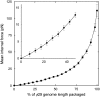
Similar articles
-
Ionic effects on viral DNA packaging and portal motor function in bacteriophage phi 29.Proc Natl Acad Sci U S A. 2007 Jul 3;104(27):11245-50. doi: 10.1073/pnas.0701323104. Epub 2007 Jun 7. Proc Natl Acad Sci U S A. 2007. PMID: 17556543 Free PMC article.
-
Experimental comparison of forces resisting viral DNA packaging and driving DNA ejection.Phys Rev E. 2017 May;95(5-1):052408. doi: 10.1103/PhysRevE.95.052408. Epub 2017 May 17. Phys Rev E. 2017. PMID: 28618627 Free PMC article.
-
Measurements of single DNA molecule packaging dynamics in bacteriophage lambda reveal high forces, high motor processivity, and capsid transformations.J Mol Biol. 2007 Nov 9;373(5):1113-22. doi: 10.1016/j.jmb.2007.09.011. Epub 2007 Sep 11. J Mol Biol. 2007. PMID: 17919653 Free PMC article.
-
Construction of bacteriophage phi29 DNA packaging motor and its applications in nanotechnology and therapy.Ann Biomed Eng. 2009 Oct;37(10):2064-81. doi: 10.1007/s10439-009-9723-0. Epub 2009 Jun 4. Ann Biomed Eng. 2009. PMID: 19495981 Free PMC article. Review.
-
Bacterial virus phi29 DNA-packaging motor and its potential applications in gene therapy and nanotechnology.Methods Mol Biol. 2005;300:285-324. doi: 10.1385/1-59259-858-7:285. Methods Mol Biol. 2005. PMID: 15657489 Review.
Cited by
-
Condensed genome structure.Adv Exp Med Biol. 2012;726:469-87. doi: 10.1007/978-1-4614-0980-9_21. Adv Exp Med Biol. 2012. PMID: 22297527 Free PMC article. Review.
-
Insights into a viral motor: the structure of the HK97 packaging termination assembly.Nucleic Acids Res. 2023 Jul 21;51(13):7025-7035. doi: 10.1093/nar/gkad480. Nucleic Acids Res. 2023. PMID: 37293963 Free PMC article.
-
Structural ensemble and dynamics of toroidal-like DNA shapes in bacteriophage ϕ29 exit cavity.Biophys J. 2013 May 7;104(9):2058-67. doi: 10.1016/j.bpj.2013.03.032. Biophys J. 2013. PMID: 23663849 Free PMC article.
-
Intersubunit coordination in a homomeric ring ATPase.Nature. 2009 Jan 22;457(7228):446-50. doi: 10.1038/nature07637. Epub 2009 Jan 7. Nature. 2009. PMID: 19129763 Free PMC article.
-
Controlled Mechanical Motions of Microparticles in Optical Tweezers.Micromachines (Basel). 2018 May 12;9(5):232. doi: 10.3390/mi9050232. Micromachines (Basel). 2018. PMID: 30424165 Free PMC article. Review.
References
-
- Jardine, P. J., and D. Anderson. 2006. DNA packaging in double-stranded DNA bacteriophages. In The Bacteriophages. R. Calendar, editor. Oxford University Press, Oxford, UK.
-
- Grimes, S., P. J. Jardine, and D. Anderson. 2002. Bacteriophage φ29 DNA packaging. Adv. Virus Res. 58:255–294. - PubMed
-
- Smith, D. E., S. J. Tans, S. B. Smith, S. Grimes, D. L. Anderson, and C. Bustamante. 2001. The bacteriophage φ29 portal motor can package DNA against a large internal force. Nature. 413:748–752. - PubMed
-
- Guasch, A., J. Pous, B. Ibarra, F. X. Gomis-Ruth, J. M. Valpuesta, N. Sousa, J. L. Carrascosa, and M. Coll. 2002. Detailed architecture of a DNA translocating machine: the high-resolution structure of the bacteriophage φ29 connector particle. J. Mol. Biol. 315:663–676. - PubMed
Publication types
MeSH terms
Substances
Grants and funding
LinkOut - more resources
Full Text Sources