Nuclear magnetic resonance structure of the N-terminal domain of nonstructural protein 3 from the severe acute respiratory syndrome coronavirus
- PMID: 17728234
- PMCID: PMC2168779
- DOI: 10.1128/JVI.00969-07
Nuclear magnetic resonance structure of the N-terminal domain of nonstructural protein 3 from the severe acute respiratory syndrome coronavirus
Abstract
This paper describes the structure determination of nsp3a, the N-terminal domain of the severe acute respiratory syndrome coronavirus (SARS-CoV) nonstructural protein 3. nsp3a exhibits a ubiquitin-like globular fold of residues 1 to 112 and a flexibly extended glutamic acid-rich domain of residues 113 to 183. In addition to the four beta-strands and two alpha-helices that are common to ubiquitin-like folds, the globular domain of nsp3a contains two short helices representing a feature that has not previously been observed in these proteins. Nuclear magnetic resonance chemical shift perturbations showed that these unique structural elements are involved in interactions with single-stranded RNA. Structural similarities with proteins involved in various cell-signaling pathways indicate possible roles of nsp3a in viral infection and persistence.
Figures
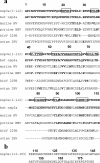
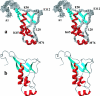
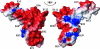
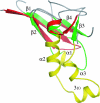
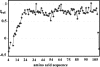
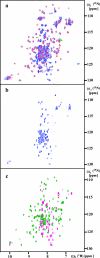
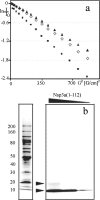
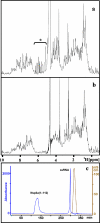
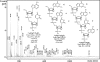
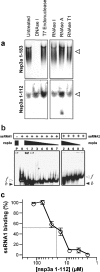
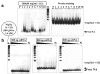
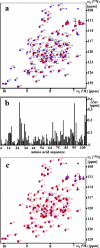
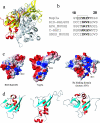
Similar articles
-
Nuclear magnetic resonance structure shows that the severe acute respiratory syndrome coronavirus-unique domain contains a macrodomain fold.J Virol. 2009 Feb;83(4):1823-36. doi: 10.1128/JVI.01781-08. Epub 2008 Dec 3. J Virol. 2009. PMID: 19052085 Free PMC article.
-
Nuclear magnetic resonance structure of the nucleic acid-binding domain of severe acute respiratory syndrome coronavirus nonstructural protein 3.J Virol. 2009 Dec;83(24):12998-3008. doi: 10.1128/JVI.01253-09. Epub 2009 Oct 14. J Virol. 2009. PMID: 19828617 Free PMC article.
-
Novel beta-barrel fold in the nuclear magnetic resonance structure of the replicase nonstructural protein 1 from the severe acute respiratory syndrome coronavirus.J Virol. 2007 Apr;81(7):3151-61. doi: 10.1128/JVI.01939-06. Epub 2007 Jan 3. J Virol. 2007. PMID: 17202208 Free PMC article.
-
Nsp3 of coronaviruses: Structures and functions of a large multi-domain protein.Antiviral Res. 2018 Jan;149:58-74. doi: 10.1016/j.antiviral.2017.11.001. Epub 2017 Nov 8. Antiviral Res. 2018. PMID: 29128390 Free PMC article. Review.
-
SARS coronavirus accessory proteins.Virus Res. 2008 Apr;133(1):113-21. doi: 10.1016/j.virusres.2007.10.009. Epub 2007 Nov 28. Virus Res. 2008. PMID: 18045721 Free PMC article. Review.
Cited by
-
Molecular mechanisms of human coronavirus NL63 infection and replication.Virus Res. 2023 Apr 2;327:199078. doi: 10.1016/j.virusres.2023.199078. Epub 2023 Feb 22. Virus Res. 2023. PMID: 36813239 Free PMC article. Review.
-
Structural insights into ribonucleoprotein dissociation by nucleocapsid protein interacting with non-structural protein 3 in SARS-CoV-2.Commun Biol. 2023 Feb 18;6(1):193. doi: 10.1038/s42003-023-04570-2. Commun Biol. 2023. PMID: 36806252 Free PMC article.
-
Humoral Immune Response Profile of COVID-19 Reveals Severity and Variant-Specific Epitopes: Lessons from SARS-CoV-2 Peptide Microarray.Viruses. 2023 Jan 15;15(1):248. doi: 10.3390/v15010248. Viruses. 2023. PMID: 36680289 Free PMC article.
-
Molecular Dynamics Simulations to Decipher the Role of Phosphorylation of SARS-CoV-2 Nonstructural Proteins (nsps) in Viral Replication.Viruses. 2022 Nov 2;14(11):2436. doi: 10.3390/v14112436. Viruses. 2022. PMID: 36366534 Free PMC article.
-
Unique mutations in SARS-CoV-2 Omicron subvariants' non-spike proteins: Potential impacts on viral pathogenesis and host immune evasion.Microb Pathog. 2022 Sep;170:105699. doi: 10.1016/j.micpath.2022.105699. Epub 2022 Aug 6. Microb Pathog. 2022. PMID: 35944840 Free PMC article. Review.
References
-
- Altieri, A. S., D. P. Hinton, and R. A. Byrd. 1995. Association of biomolecular systems via pulsed field gradient NMR self-diffusion measurements. J. Am. Chem. Soc. 117:7566-7567.
-
- Cornell, W. D., P. Cieplak, C. I. Bayly, I. R. Gould, J. Merz, K. M., D. M. Ferguson, D. C. Spellmyer, T. Fox, J. W. Caldwell, and P. A. Kollman. 1995. A second generation force field for the simulation of proteins, nucleic acids, and organic molecules. J. Am. Chem. Soc. 117:5179-5197.
Publication types
MeSH terms
Substances
Associated data
- Actions
- Actions
Grants and funding
LinkOut - more resources
Full Text Sources
Miscellaneous