A WD40 domain cyclophilin interacts with histone H3 and functions in gene repression and organogenesis in Arabidopsis
- PMID: 17704213
- PMCID: PMC2002612
- DOI: 10.1105/tpc.107.053579
A WD40 domain cyclophilin interacts with histone H3 and functions in gene repression and organogenesis in Arabidopsis
Abstract
Chromatin-based silencing provides a crucial mechanism for the regulation of gene expression. We have identified a WD40 domain cyclophilin, CYCLOPHILIN71 (CYP71), which functions in gene repression and organogenesis in Arabidopsis thaliana. Disruption of CYP71 resulted in ectopic activation of homeotic genes that regulate meristem development. The cyp71 mutant plants displayed dramatic defects, including reduced apical meristem activity, delayed and abnormal lateral organ formation, and arrested root growth. CYP71 was associated with the chromatin of target gene loci and physically interacted with histone H3. The cyp71 mutant showed reduced methylation of H3K27 at target loci, consistent with the derepression of these genes in the mutant. As CYP71 has close homologs in eukaryotes ranging from fission yeast to human, we propose that it serves as a highly conserved histone remodeling factor involved in chromatin-based gene silencing in eukaryotic organisms.
Figures
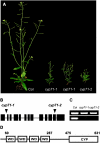
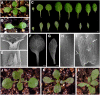
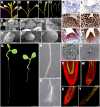
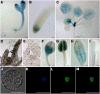
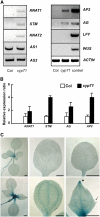
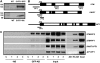
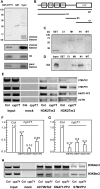
Similar articles
-
Expression analysis of Arabidopsis XH/XS-domain proteins indicates overlapping and distinct functions for members of this gene family.J Exp Bot. 2014 Mar;65(4):1217-27. doi: 10.1093/jxb/ert480. J Exp Bot. 2014. PMID: 24574485 Free PMC article.
-
Depressing time: Waiting, melancholia, and the psychoanalytic practice of care.In: Kirtsoglou E, Simpson B, editors. The Time of Anthropology: Studies of Contemporary Chronopolitics. Abingdon: Routledge; 2020. Chapter 5. In: Kirtsoglou E, Simpson B, editors. The Time of Anthropology: Studies of Contemporary Chronopolitics. Abingdon: Routledge; 2020. Chapter 5. PMID: 36137063 Free Books & Documents. Review.
-
Characterization of the Arabidopsis thaliana chromatin remodeler DEK3 for its interaction with histones and DNA.Biochimie. 2024 Dec;227(Pt A):248-261. doi: 10.1016/j.biochi.2024.07.018. Epub 2024 Aug 6. Biochimie. 2024. PMID: 39097158
-
SUPPRESSOR OF FRIGIDA 4 cooperates with the histone methylation reader EBS to positively regulate root development.Plant Physiol. 2024 Dec 2;196(4):2199-2212. doi: 10.1093/plphys/kiae321. Plant Physiol. 2024. PMID: 38875008
-
Topical fluoride as a cause of dental fluorosis in children.Cochrane Database Syst Rev. 2024 Jun 20;6(6):CD007693. doi: 10.1002/14651858.CD007693.pub3. Cochrane Database Syst Rev. 2024. PMID: 38899538 Review.
Cited by
-
Versatility of Cyclophilins in Plant Growth and Survival: A Case Study in Arabidopsis.Biomolecules. 2019 Jan 10;9(1):20. doi: 10.3390/biom9010020. Biomolecules. 2019. PMID: 30634678 Free PMC article. Review.
-
Unique N-Terminal Interactions Connect F-BOX STRESS INDUCED (FBS) Proteins to a WD40 Repeat-like Protein Pathway in Arabidopsis.Plants (Basel). 2021 Oct 19;10(10):2228. doi: 10.3390/plants10102228. Plants (Basel). 2021. PMID: 34686037 Free PMC article.
-
Cyclophilins and Their Functions in Abiotic Stress and Plant-Microbe Interactions.Biomolecules. 2021 Sep 21;11(9):1390. doi: 10.3390/biom11091390. Biomolecules. 2021. PMID: 34572603 Free PMC article. Review.
-
Positive selection and heat-response transcriptomes reveal adaptive features of the Brassicaceae desert model, Anastatica hierochuntica.New Phytol. 2022 Nov;236(3):1006-1026. doi: 10.1111/nph.18411. Epub 2022 Aug 26. New Phytol. 2022. PMID: 35909295 Free PMC article.
-
OsLIS-L1 encoding a lissencephaly type-1-like protein with WD40 repeats is required for plant height and male gametophyte formation in rice.Planta. 2012 Apr;235(4):713-27. doi: 10.1007/s00425-011-1532-7. Epub 2011 Oct 22. Planta. 2012. PMID: 22020753
References
-
- Alonso, J.M., et al. (2003). Genome-wide insertional mutagenesis of Arabidopsis thaliana. Science 301 653–657. - PubMed
-
- Berardini, T.Z., Bollman, K., Sun, H., and Poethig, R.S. (2001). Regulation of vegetative phase change in Arabidopsis thaliana by cyclophilin 40. Science 291 2405–2407. - PubMed
-
- Berger, F., and Gaudin, V. (2003). Chromatin dynamics and Arabidopsis development. Chromosome Res. 11 277–304. - PubMed
-
- Berger, S.L. (2007). The complex language of chromatin regulation during transcription. Nature 447 407–412. - PubMed
-
- Beuchle, D., Struhl, G., and Muller, J. (2001). Polycomb group proteins and heritable silencing of Drosophila Hox genes. Development 128 993–1004. - PubMed
Publication types
MeSH terms
Substances
LinkOut - more resources
Full Text Sources
Molecular Biology Databases
Research Materials