An in vitro nuclear disassembly system reveals a role for the RanGTPase system and microtubule-dependent steps in nuclear envelope breakdown
- PMID: 17698605
- PMCID: PMC2064467
- DOI: 10.1083/jcb.200703002
An in vitro nuclear disassembly system reveals a role for the RanGTPase system and microtubule-dependent steps in nuclear envelope breakdown
Abstract
During prophase, vertebrate cells disassemble their nuclear envelope (NE) in the process of NE breakdown (NEBD). We have established an in vitro assay that uses mitotic Xenopus laevis egg extracts and semipermeabilized somatic cells bearing a green fluorescent protein-tagged NE marker to study the molecular requirements underlying the dynamic changes of the NE during NEBD by live microscopy. We applied our in vitro system to analyze the role of the Ran guanosine triphosphatase (GTPase) system in NEBD. Our study shows that high levels of RanGTP affect the dynamics of late steps of NEBD in vitro. Also, inhibition of RanGTP production by RanT24N blocks the dynamic rupture of nuclei, suggesting that the local generation of RanGTP around chromatin may serve as a spatial cue in NEBD. Furthermore, the microtubule-depolymerizing drug nocodazole interferes with late steps of nuclear disassembly in vitro. High resolution live cell imaging reveals that microtubules are involved in the completion of NEBD in vivo by facilitating the efficient removal of membranes from chromatin.
Figures
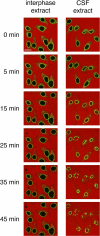
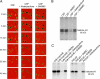
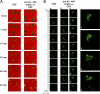
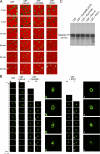
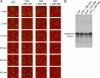
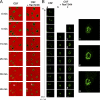
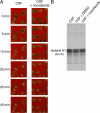
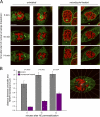
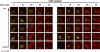
Similar articles
-
Analysis of a RanGTP-regulated gradient in mitotic somatic cells.Nature. 2006 Mar 30;440(7084):697-701. doi: 10.1038/nature04589. Nature. 2006. PMID: 16572176
-
Characterization of Ran-driven cargo transport and the RanGTPase system by kinetic measurements and computer simulation.EMBO J. 2003 Mar 3;22(5):1088-100. doi: 10.1093/emboj/cdg113. EMBO J. 2003. PMID: 12606574 Free PMC article.
-
An in vitro system to study nuclear envelope breakdown.Methods Cell Biol. 2014;122:255-76. doi: 10.1016/B978-0-12-417160-2.00012-6. Methods Cell Biol. 2014. PMID: 24857734
-
Spatial and temporal control of nuclear envelope assembly by Ran GTPase.Symp Soc Exp Biol. 2004;(56):193-204. Symp Soc Exp Biol. 2004. PMID: 15565882 Review.
-
Composition of the plant nuclear envelope: theme and variations.J Exp Bot. 2007;58(1):27-34. doi: 10.1093/jxb/erl009. Epub 2006 Jun 27. J Exp Bot. 2007. PMID: 16804054 Review.
Cited by
-
Nucleus-Cytoskeleton Crosstalk During Mitotic Entry.Front Cell Dev Biol. 2021 Mar 18;9:649899. doi: 10.3389/fcell.2021.649899. eCollection 2021. Front Cell Dev Biol. 2021. PMID: 33816500 Free PMC article. Review.
-
Temporal control of nuclear envelope assembly by phosphorylation of lamin B receptor.Mol Biol Cell. 2011 Sep;22(18):3306-17. doi: 10.1091/mbc.E11-03-0199. Epub 2011 Jul 27. Mol Biol Cell. 2011. PMID: 21795390 Free PMC article.
-
Phosphorylation of nucleoporin Tpr governs its differential localization and is required for its mitotic function.J Cell Sci. 2014 Aug 15;127(Pt 16):3505-20. doi: 10.1242/jcs.149112. Epub 2014 Jun 17. J Cell Sci. 2014. PMID: 24938596 Free PMC article.
-
Chromosome segregation errors generate a diverse spectrum of simple and complex genomic rearrangements.Nat Genet. 2019 Apr;51(4):705-715. doi: 10.1038/s41588-019-0360-8. Epub 2019 Mar 4. Nat Genet. 2019. PMID: 30833795 Free PMC article.
-
Double duty for nuclear proteins--the price of more open forms of mitosis.Trends Genet. 2009 Dec;25(12):545-54. doi: 10.1016/j.tig.2009.10.005. Epub 2009 Oct 29. Trends Genet. 2009. PMID: 19879010 Free PMC article. Review.
References
-
- Beaudouin, J., D. Gerlich, N. Daigle, R. Eils, and J. Ellenberg. 2002. Nuclear envelope breakdown proceeds by microtubule-induced tearing of the lamina. Cell. 108:83–96. - PubMed
-
- Busson, S., D. Dujardin, A. Moreau, J. Dompierre, and J.R. De Mey. 1998. Dynein and dynactin are localized to astral microtubules and at cortical sites in mitotic epithelial cells. Curr. Biol. 8:541–544. - PubMed
-
- Carazo-Salas, R.E., G. Guarguaglini, O.J. Gruss, A. Segref, E. Karsenti, and I.W. Mattaj. 1999. Generation of GTP-bound Ran by RCC1 is required for chromatin-induced mitotic spindle formation. Nature. 400:178–181. - PubMed
Publication types
MeSH terms
Substances
LinkOut - more resources
Full Text Sources
Other Literature Sources
Miscellaneous