An ACAP1-containing clathrin coat complex for endocytic recycling
- PMID: 17664335
- PMCID: PMC2064835
- DOI: 10.1083/jcb.200608033
An ACAP1-containing clathrin coat complex for endocytic recycling
Abstract
Whether coat proteins play a widespread role in endocytic recycling remains unclear. We find that ACAP1, a GTPase-activating protein (GAP) for ADP-ribosylation factor (ARF) 6, is part of a novel clathrin coat complex that is regulated by ARF6 for endocytic recycling in two key physiological settings, stimulation-dependent recycling of integrin that is critical for cell migration and insulin-stimulated recycling of glucose transporter type 4 (Glut4), which is required for glucose homeostasis. These findings not only advance a basic understanding of an early mechanistic step in endocytic recycling but also shed key mechanistic insights into major physiological events for which this transport plays a critical role.
Figures
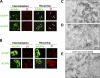
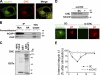
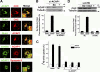
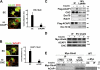
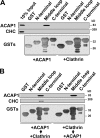
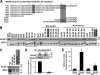
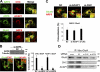
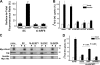
Similar articles
-
An ACAP1 coat complex acting in endocytic recycling.Methods Cell Biol. 2015;130:81-99. doi: 10.1016/bs.mcb.2015.03.019. Epub 2015 Jun 11. Methods Cell Biol. 2015. PMID: 26360030
-
A novel GTPase-activating protein for ARF6 directly interacts with clathrin and regulates clathrin-dependent endocytosis.Mol Biol Cell. 2005 Apr;16(4):1617-28. doi: 10.1091/mbc.e04-08-0683. Epub 2005 Jan 19. Mol Biol Cell. 2005. PMID: 15659652 Free PMC article.
-
Mechanistic insights into regulated cargo binding by ACAP1 protein.J Biol Chem. 2012 Aug 17;287(34):28675-85. doi: 10.1074/jbc.M112.378810. Epub 2012 May 29. J Biol Chem. 2012. PMID: 22645133 Free PMC article.
-
Arf GAPs: A family of proteins with disparate functions that converge on a common structure, the integrin adhesion complex.Small GTPases. 2019 Jul;10(4):280-288. doi: 10.1080/21541248.2017.1299271. Epub 2017 Mar 31. Small GTPases. 2019. PMID: 28362242 Free PMC article. Review.
-
GRAF1-dependent endocytosis.Biochem Soc Trans. 2009 Oct;37(Pt 5):1061-5. doi: 10.1042/BST0371061. Biochem Soc Trans. 2009. PMID: 19754452 Review.
Cited by
-
Arf GAPs and molecular motors.Small GTPases. 2019 May;10(3):196-209. doi: 10.1080/21541248.2017.1308850. Epub 2017 Apr 21. Small GTPases. 2019. PMID: 28430047 Free PMC article. Review.
-
Akt may associate with insulin-responsive vesicles via interaction with sortilin.FEBS Lett. 2024 Feb;598(4):390-399. doi: 10.1002/1873-3468.14790. Epub 2023 Dec 21. FEBS Lett. 2024. PMID: 38105115
-
Clathrin and AP1B: key roles in basolateral trafficking through trans-endosomal routes.FEBS Lett. 2009 Dec 3;583(23):3784-95. doi: 10.1016/j.febslet.2009.10.050. Epub 2009 Oct 23. FEBS Lett. 2009. PMID: 19854182 Free PMC article. Review.
-
Exclusion of integrins from CNS axons is regulated by Arf6 activation and the AIS.J Neurosci. 2015 May 27;35(21):8359-75. doi: 10.1523/JNEUROSCI.2850-14.2015. J Neurosci. 2015. PMID: 26019348 Free PMC article.
-
RGD-Binding Integrins Revisited: How Recently Discovered Functions and Novel Synthetic Ligands (Re-)Shape an Ever-Evolving Field.Cancers (Basel). 2021 Apr 4;13(7):1711. doi: 10.3390/cancers13071711. Cancers (Basel). 2021. PMID: 33916607 Free PMC article. Review.
References
-
- Balañá, M.E., F. Niedergang, A. Subtil, A. Alcover, P. Chavrier, and A. Daultry-Varsat. 2005. ARF6 GTPase controls bacterial invasion by actin remodelling. J. Cell Sci. 118:2201–2210. - PubMed
-
- Barlowe, C., L. Orci, T. Yeung, M. Hosobuchi, S. Hamamoto, N. Salama, M.F. Rexach, M. Ravazzola, M. Amherdt, and R. Schekman. 1994. COPII: a membrane coat formed by Sec proteins that drive vesicle budding from the endoplasmic reticulum. Cell. 77:895–907. - PubMed
-
- Bogan, J.S., N. Hendon, A.E. McKee, T.S. Tsao, and H.F. Lodish. 2003. Functional cloning of TUG as a regulator of GLUT4 glucose transporter trafficking. Nature. 425:727–733. - PubMed
Publication types
MeSH terms
Substances
Grants and funding
LinkOut - more resources
Full Text Sources
Other Literature Sources
Molecular Biology Databases
Miscellaneous