Genome-wide analyses reveal properties of redundant and specific promoter occupancy within the ETS gene family
- PMID: 17652178
- PMCID: PMC1935027
- DOI: 10.1101/gad.1561707
Genome-wide analyses reveal properties of redundant and specific promoter occupancy within the ETS gene family
Abstract
The conservation of in vitro DNA-binding properties within families of transcription factors presents a challenge for achieving in vivo specificity. To uncover the mechanisms regulating specificity within the ETS gene family, we have used chromatin immunoprecipitation coupled with genome-wide promoter microarrays to query the occupancy of three ETS proteins in a human T-cell line. Unexpectedly, redundant occupancy was frequently detected, while specific occupancy was less likely. Redundant binding correlated with housekeeping classes of genes, whereas specific binding examples represented more specialized genes. Bioinformatics approaches demonstrated that redundant binding correlated with consensus ETS-binding sequences near transcription start sites. In contrast, specific binding sites diverged dramatically from the consensus and were found further from transcription start sites. One route to specificity was found--a highly divergent binding site that facilitates ETS1 and RUNX1 cooperative DNA binding. The specific and redundant DNA-binding modes suggest two distinct roles for members of the ETS transcription factor family.
Figures
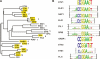
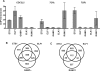
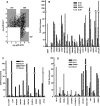
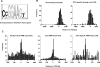
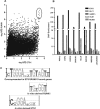
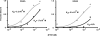
Similar articles
-
DNA specificity determinants associate with distinct transcription factor functions.PLoS Genet. 2009 Dec;5(12):e1000778. doi: 10.1371/journal.pgen.1000778. Epub 2009 Dec 18. PLoS Genet. 2009. PMID: 20019798 Free PMC article.
-
Regulation of the human leukemia inhibitory factor gene by ETS transcription factors.Neuroimmunomodulation. 2004;11(1):10-9. doi: 10.1159/000072964. Neuroimmunomodulation. 2004. PMID: 14557674
-
The human CD6 gene is transcriptionally regulated by RUNX and Ets transcription factors in T cells.Mol Immunol. 2009 Jul;46(11-12):2226-35. doi: 10.1016/j.molimm.2009.04.018. Epub 2009 May 14. Mol Immunol. 2009. PMID: 19446338
-
Genomic and biochemical insights into the specificity of ETS transcription factors.Annu Rev Biochem. 2011;80:437-71. doi: 10.1146/annurev.biochem.79.081507.103945. Annu Rev Biochem. 2011. PMID: 21548782 Free PMC article. Review.
-
Molecular analysis of the ets genes and their products.Crit Rev Oncog. 1990;1(4):409-36. Crit Rev Oncog. 1990. PMID: 1964597 Review.
Cited by
-
Ets1 and Elk1 transcription factors regulate cancerous inhibitor of protein phosphatase 2A expression in cervical and endometrial carcinoma cells.Transcription. 2012 Nov-Dec;3(6):323-35. doi: 10.4161/trns.22518. Epub 2012 Nov 1. Transcription. 2012. PMID: 23117818 Free PMC article.
-
The ETS Family Transcription Factors Etv5 and PU.1 Function in Parallel To Promote Th9 Cell Development.J Immunol. 2016 Sep 15;197(6):2465-72. doi: 10.4049/jimmunol.1502383. Epub 2016 Aug 5. J Immunol. 2016. PMID: 27496971 Free PMC article.
-
A quartet of PIF bHLH factors provides a transcriptionally centered signaling hub that regulates seedling morphogenesis through differential expression-patterning of shared target genes in Arabidopsis.PLoS Genet. 2013;9(1):e1003244. doi: 10.1371/journal.pgen.1003244. Epub 2013 Jan 31. PLoS Genet. 2013. PMID: 23382695 Free PMC article.
-
Opposing functions of the ETS factor family define Shh spatial expression in limb buds and underlie polydactyly.Dev Cell. 2012 Feb 14;22(2):459-67. doi: 10.1016/j.devcel.2011.12.010. Dev Cell. 2012. PMID: 22340503 Free PMC article.
-
Elucidation of the ELK1 target gene network reveals a role in the coordinate regulation of core components of the gene regulation machinery.Genome Res. 2009 Nov;19(11):1963-73. doi: 10.1101/gr.093047.109. Epub 2009 Aug 17. Genome Res. 2009. PMID: 19687146 Free PMC article.
References
-
- Aerts S., Van Loo P., Thijs G., Moreau Y., De Moor B., Van Loo P., Thijs G., Moreau Y., De Moor B., Thijs G., Moreau Y., De Moor B., Moreau Y., De Moor B., De Moor B. Computational detection of cis-regulatory modules. Bioinformatics. 2003;19 (Suppl. 2):II5–II14. - PubMed
-
- Bailey T.L., Elkan C., Elkan C. Fitting a mixture model by expectation maximization to discover motifs in biopolymers. In: Altman R., et al., editors. Proceedings of the second international conference on intelligent systems for molecular biology. AAAI Press; Menlo Park, CA: 1994. pp. 28–36. - PubMed
-
- Batchelor A.H., Piper D.E., de la Brousse F.C., McKnight S.L., Wolberger C., Piper D.E., de la Brousse F.C., McKnight S.L., Wolberger C., de la Brousse F.C., McKnight S.L., Wolberger C., McKnight S.L., Wolberger C., Wolberger C. The structure of GABPα/β: An ETS domain–ankyrin repeat heterodimer bound to DNA. Science. 1998;279:1037–1041. - PubMed
-
- Beissbarth T., Speed T.P., Speed T.P. GOstat: Find statistically overrepresented Gene Ontologies within a group of genes. Bioinformatics. 2004;20:1464–1465. - PubMed
-
- Bina M., Wyss P., Ren W., Szpankowski W., Thomas E., Randhawa R., Reddy S., John P.M., Pares-Matos E.I., Stein A., Wyss P., Ren W., Szpankowski W., Thomas E., Randhawa R., Reddy S., John P.M., Pares-Matos E.I., Stein A., Ren W., Szpankowski W., Thomas E., Randhawa R., Reddy S., John P.M., Pares-Matos E.I., Stein A., Szpankowski W., Thomas E., Randhawa R., Reddy S., John P.M., Pares-Matos E.I., Stein A., Thomas E., Randhawa R., Reddy S., John P.M., Pares-Matos E.I., Stein A., Randhawa R., Reddy S., John P.M., Pares-Matos E.I., Stein A., Reddy S., John P.M., Pares-Matos E.I., Stein A., John P.M., Pares-Matos E.I., Stein A., Pares-Matos E.I., Stein A., Stein A., et al. Exploring the characteristics of sequence elements in proximal promoters of human genes. Genomics. 2004;84:929–940. - PubMed
Publication types
MeSH terms
Substances
Grants and funding
LinkOut - more resources
Full Text Sources
Other Literature Sources
Molecular Biology Databases
Miscellaneous