A novel testis ubiquitin-binding protein gene arose by exon shuffling in hominoids
- PMID: 17623810
- PMCID: PMC1933510
- DOI: 10.1101/gr.6252107
A novel testis ubiquitin-binding protein gene arose by exon shuffling in hominoids
Abstract
Most new genes arise by duplication of existing gene structures, after which relaxed selection on the new copy frequently leads to mutational inactivation of the duplicate; only rarely will a new gene with modified function emerge. Here we describe a unique mechanism of gene creation, whereby new combinations of functional domains are assembled at the RNA level from distinct genes, and the resulting chimera is then reverse transcribed and integrated into the genome by the L1 retrotransposon. We characterized a novel gene, which we termed PIP5K1A and PSMD4-like (PIPSL), created by this mechanism from an intergenic transcript between the phosphatidylinositol-4-phosphate 5-kinase (PIP5K1A) and the 26S proteasome subunit (PSMD4) genes in a hominoid ancestor. PIPSL is transcribed specifically in the testis both in humans and chimpanzees, and is post-transcriptionally repressed by independent mechanisms in these primate lineages. The PIPSL gene encodes a chimeric protein combining the lipid kinase domain of PIP5K1A and the ubiquitin-binding motifs of PSMD4. Strong positive selection on PIPSL led to its rapid divergence from the parental genes PIP5K1A and PSMD4, forming a chimeric protein with a distinct cellular localization and minimal lipid kinase activity, but significant affinity for cellular ubiquitinated proteins. PIPSL is a tightly regulated, testis-specific novel ubiquitin-binding protein formed by an unusual exon-shuffling mechanism in hominoid primates and represents a key example of rapid evolution of a testis-specific gene.
Figures

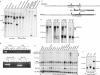
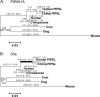
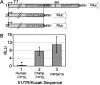
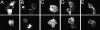
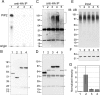
Similar articles
-
Inference for the initial stage of domain shuffling: tracing the evolutionary fate of the PIPSL retrogene in hominoids.Mol Biol Evol. 2010 Nov;27(11):2522-33. doi: 10.1093/molbev/msq138. Epub 2010 Jun 4. Mol Biol Evol. 2010. PMID: 20525901
-
Positive selection for the male functionality of a co-retroposed gene in the hominoids.BMC Evol Biol. 2009 Oct 15;9:252. doi: 10.1186/1471-2148-9-252. BMC Evol Biol. 2009. PMID: 19832993 Free PMC article.
-
Transcriptional activation of a chimeric retrogene PIPSL in a hominoid ancestor.Gene. 2018 Dec 15;678:318-323. doi: 10.1016/j.gene.2018.08.033. Epub 2018 Aug 8. Gene. 2018. PMID: 30096459
-
Ubiquitin-binding domains.Nat Rev Mol Cell Biol. 2005 Aug;6(8):610-21. doi: 10.1038/nrm1701. Nat Rev Mol Cell Biol. 2005. PMID: 16064137 Review.
-
Origin of new genes: evidence from experimental and computational analyses.Genetica. 2003 Jul;118(2-3):171-82. Genetica. 2003. PMID: 12868607 Review.
Cited by
-
Quantifying the mechanisms of domain gain in animal proteins.Genome Biol. 2010;11(7):R74. doi: 10.1186/gb-2010-11-7-r74. Epub 2010 Jul 15. Genome Biol. 2010. PMID: 20633280 Free PMC article.
-
Restless genomes humans as a model organism for understanding host-retrotransposable element dynamics.Adv Genet. 2011;73:219-62. doi: 10.1016/B978-0-12-380860-8.00006-9. Adv Genet. 2011. PMID: 21310298 Free PMC article. Review.
-
Expression of evolutionarily novel genes in tumors.Infect Agent Cancer. 2016 Jul 19;11:34. doi: 10.1186/s13027-016-0077-6. eCollection 2016. Infect Agent Cancer. 2016. PMID: 27437030 Free PMC article. Review.
-
Adaptive impact of the chimeric gene Quetzalcoatl in Drosophila melanogaster.Proc Natl Acad Sci U S A. 2010 Jun 15;107(24):10943-8. doi: 10.1073/pnas.1006503107. Epub 2010 Jun 1. Proc Natl Acad Sci U S A. 2010. PMID: 20534482 Free PMC article.
-
It Is Imperative to Establish a Pellucid Definition of Chimeric RNA and to Clear Up a Lot of Confusion in the Relevant Research.Int J Mol Sci. 2017 Mar 28;18(4):714. doi: 10.3390/ijms18040714. Int J Mol Sci. 2017. PMID: 28350330 Free PMC article. Review.
References
-
- Akiva P., Toporik A., Edelheit S., Peretz Y., Diber A., Shemesh R., Novik A., Sorek R., Toporik A., Edelheit S., Peretz Y., Diber A., Shemesh R., Novik A., Sorek R., Edelheit S., Peretz Y., Diber A., Shemesh R., Novik A., Sorek R., Peretz Y., Diber A., Shemesh R., Novik A., Sorek R., Diber A., Shemesh R., Novik A., Sorek R., Shemesh R., Novik A., Sorek R., Novik A., Sorek R., Sorek R. Transcription-mediated gene fusion in the human genome. Genome Res. 2006;16:30–36. - PMC - PubMed
-
- Anand G., Yin X., Shahidi A.K., Grove L., Prochownik E.V., Yin X., Shahidi A.K., Grove L., Prochownik E.V., Shahidi A.K., Grove L., Prochownik E.V., Grove L., Prochownik E.V., Prochownik E.V. Novel regulation of the helix–loop–helix protein Id1 by S5a, a subunit of the 26 S proteasome. J. Biol. Chem. 1997;272:19140–19151. - PubMed
-
- Arioka M., Nakashima S., Shibasaki Y., Kitamoto K., Nakashima S., Shibasaki Y., Kitamoto K., Shibasaki Y., Kitamoto K., Kitamoto K. Dibasic amino acid residues at the carboxy-terminal end of kinase homology domain participate in the plasma membrane localization and function of phosphatidylinositol 5-kinase gamma. Biochem. Biophys. Res. Commun. 2004;319:456–463. - PubMed
-
- Babushok D.V., Kazazian H.H., Kazazian H.H.2007Progress in understanding the biology of the human mutagen LINE-1 Hum. Mutat. 28 :527–539. - PubMed
Publication types
MeSH terms
Substances
LinkOut - more resources
Full Text Sources
Molecular Biology Databases