Reconstructing the evolution of the mitochondrial ribosomal proteome
- PMID: 17604309
- PMCID: PMC1950548
- DOI: 10.1093/nar/gkm441
Reconstructing the evolution of the mitochondrial ribosomal proteome
Abstract
For production of proteins that are encoded by the mitochondrial genome, mitochondria rely on their own mitochondrial translation system, with the mitoribosome as its central component. Using extensive homology searches, we have reconstructed the evolutionary history of the mitoribosomal proteome that is encoded by a diverse subset of eukaryotic genomes, revealing an ancestral ribosome of alpha-proteobacterial descent that more than doubled its protein content in most eukaryotic lineages. We observe large variations in the protein content of mitoribosomes between different eukaryotes, with mammalian mitoribosomes sharing only 74 and 43% of its proteins with yeast and Leishmania mitoribosomes, respectively. We detected many previously unidentified mitochondrial ribosomal proteins (MRPs) and found that several have increased in size compared to their bacterial ancestral counterparts by addition of functional domains. Several new MRPs have originated via duplication of existing MRPs as well as by recruitment from outside of the mitoribosomal proteome. Using sensitive profile-profile homology searches, we found hitherto undetected homology between bacterial and eukaryotic ribosomal proteins, as well as between fungal and mammalian ribosomal proteins, detecting two novel human MRPs. These newly detected MRPs constitute, along with evolutionary conserved MRPs, excellent new screening targets for human patients with unresolved mitochondrial oxidative phosphorylation disorders.
Figures
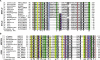
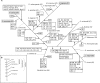
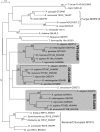
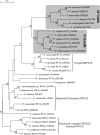
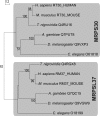
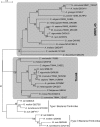
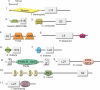
Similar articles
-
Dynamic evolution of mitochondrial ribosomal proteins in Holozoa.Mol Phylogenet Evol. 2014 Jul;76:67-74. doi: 10.1016/j.ympev.2014.03.005. Epub 2014 Mar 13. Mol Phylogenet Evol. 2014. PMID: 24631858
-
Mitochondrial ribosomal proteins (MRPs) of yeast.Biochem J. 1998 Feb 1;329 ( Pt 3)(Pt 3):433-48. doi: 10.1042/bj3290433. Biochem J. 1998. PMID: 9445368 Free PMC article. Review.
-
The gene encoding Arabidopsis thaliana mitochondrial ribosomal protein S13 is a recent duplication of the gene encoding plastid S13.Curr Genet. 2002 Mar;40(6):405-9. doi: 10.1007/s00294-002-0271-5. Epub 2002 Mar 7. Curr Genet. 2002. PMID: 11919680
-
Comparative analysis of bacterial-origin genes for plant mitochondrial ribosomal proteins.Mol Biol Evol. 2006 Mar;23(3):701-12. doi: 10.1093/molbev/msj080. Epub 2005 Dec 20. Mol Biol Evol. 2006. PMID: 16368778
-
Properties of human mitochondrial ribosomes.IUBMB Life. 2003 Sep;55(9):505-13. doi: 10.1080/15216540310001626610. IUBMB Life. 2003. PMID: 14658756 Review.
Cited by
-
Evolutionary and genetic analyses of mitochondrial translation initiation factors identify the missing mitochondrial IF3 in S. cerevisiae.Nucleic Acids Res. 2012 Jul;40(13):6122-34. doi: 10.1093/nar/gks272. Epub 2012 Mar 28. Nucleic Acids Res. 2012. PMID: 22457064 Free PMC article.
-
Enrichment of homologs in insignificant BLAST hits by co-complex network alignment.BMC Bioinformatics. 2010 Feb 12;11:86. doi: 10.1186/1471-2105-11-86. BMC Bioinformatics. 2010. PMID: 20152020 Free PMC article.
-
Cytonuclear integration and co-evolution.Nat Rev Genet. 2018 Oct;19(10):635-648. doi: 10.1038/s41576-018-0035-9. Nat Rev Genet. 2018. PMID: 30018367 Free PMC article. Review.
-
Mrpl36 is important for generation of assembly competent proteins during mitochondrial translation.Mol Biol Cell. 2009 May;20(10):2615-25. doi: 10.1091/mbc.e08-12-1162. Epub 2009 Apr 1. Mol Biol Cell. 2009. PMID: 19339279 Free PMC article.
-
Mitochondrial evolution.Cold Spring Harb Perspect Biol. 2012 Sep 1;4(9):a011403. doi: 10.1101/cshperspect.a011403. Cold Spring Harb Perspect Biol. 2012. PMID: 22952398 Free PMC article. Review.
References
-
- Gray MW, Burger G, Lang BF. Mitochondrial evolution. Science. 1999;283:1476–1481. - PubMed
-
- Margulis L. The origin of the eukaryotic cell. New Haven: Yale University Press; 1970.
-
- Saraste M. Oxidative phosphorylation at the fin de siecle. Science. 1999;283:1488–1493. - PubMed
-
- Pel HJ, Grivell LA. Protein synthesis in mitochondria. Mol. Biol. Rep. 1994;19:183–194. - PubMed
-
- Lang BF, Burger G, O’Kelly CJ, Cedergren R, Golding GB, Lemieux C, Sankoff D, Turmel M, Gray MW. An ancestral mitochondrial DNA resembling a eubacterial genome in miniature. Nature. 1997;387:493–497. - PubMed
Publication types
MeSH terms
Substances
LinkOut - more resources
Full Text Sources
Molecular Biology Databases