Impaired dopamine release and synaptic plasticity in the striatum of PINK1-deficient mice
- PMID: 17563363
- PMCID: PMC1890561
- DOI: 10.1073/pnas.0702717104
Impaired dopamine release and synaptic plasticity in the striatum of PINK1-deficient mice
Abstract
Parkinson's disease (PD) is characterized by the selective vulnerability of the nigrostriatal dopaminergic circuit. Recently, loss-of-function mutations in the PTEN-induced kinase 1 (PINK1) gene have been linked to early-onset PD. How PINK1 deficiency causes dopaminergic dysfunction and degeneration in PD patients is unknown. Here, we investigate the physiological role of PINK1 in the nigrostriatal dopaminergic circuit through the generation and multidisciplinary analysis of PINK1(-/-) mutant mice. We found that numbers of dopaminergic neurons and levels of striatal dopamine (DA) and DA receptors are unchanged in PINK1(-/-) mice. Amperometric recordings, however, revealed decreases in evoked DA release in striatal slices and reductions in the quantal size and release frequency of catecholamine in dissociated chromaffin cells. Intracellular recordings of striatal medium spiny neurons, the major dopaminergic target, showed specific impairments of corticostriatal long-term potentiation and long-term depression in PINK1(-/-) mice. Consistent with a decrease in evoked DA release, these striatal plasticity impairments could be rescued by either DA receptor agonists or agents that increase DA release, such as amphetamine or l-dopa. These results reveal a critical role for PINK1 in DA release and striatal synaptic plasticity in the nigrostriatal circuit and suggest that altered dopaminergic physiology may be a pathogenic precursor to nigrostriatal degeneration.
Conflict of interest statement
The authors declare no conflict of interest.
Figures
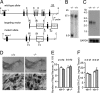
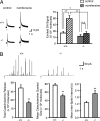
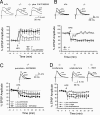
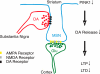
Comment in
-
Physiology versus pathology in Parkinson's disease.Proc Natl Acad Sci U S A. 2007 Jul 17;104(29):11867-8. doi: 10.1073/pnas.0704254104. Epub 2007 Jul 10. Proc Natl Acad Sci U S A. 2007. PMID: 17623781 Free PMC article. No abstract available.
Similar articles
-
Impaired dopamine release and synaptic plasticity in the striatum of parkin-/- mice.J Neurochem. 2009 Jul;110(2):613-21. doi: 10.1111/j.1471-4159.2009.06152.x. Epub 2009 May 5. J Neurochem. 2009. PMID: 19457102
-
Subtle alterations of excitatory transmission are linked to presynaptic changes in the hippocampus of PINK1-deficient mice.Synapse. 2016 Jun;70(6):223-30. doi: 10.1002/syn.21894. Epub 2016 Feb 29. Synapse. 2016. PMID: 26850695
-
Exposure to low-dose rotenone precipitates synaptic plasticity alterations in PINK1 heterozygous knockout mice.Neurobiol Dis. 2016 Jul;91:21-36. doi: 10.1016/j.nbd.2015.12.020. Epub 2016 Feb 23. Neurobiol Dis. 2016. PMID: 26916954
-
Synaptic dysfunction in Parkinson's disease.Adv Exp Med Biol. 2012;970:553-72. doi: 10.1007/978-3-7091-0932-8_24. Adv Exp Med Biol. 2012. PMID: 22351072 Review.
-
Synaptic dysfunction in Parkinson's disease.Biochem Soc Trans. 2010 Apr;38(2):493-7. doi: 10.1042/BST0380493. Biochem Soc Trans. 2010. PMID: 20298209 Review.
Cited by
-
Loss of leucine-rich repeat kinase 2 causes age-dependent bi-phasic alterations of the autophagy pathway.Mol Neurodegener. 2012 Jan 9;7:2. doi: 10.1186/1750-1326-7-2. Mol Neurodegener. 2012. PMID: 22230652 Free PMC article.
-
Mouse models of Parkinson's disease associated with mitochondrial dysfunction.Mol Cell Neurosci. 2013 Jul;55:87-94. doi: 10.1016/j.mcn.2012.08.002. Epub 2012 Aug 11. Mol Cell Neurosci. 2013. PMID: 22954895 Free PMC article. Review.
-
PINK1 and Parkin complementarily protect dopaminergic neurons in vertebrates.Hum Mol Genet. 2013 Jun 15;22(12):2423-34. doi: 10.1093/hmg/ddt095. Epub 2013 Feb 27. Hum Mol Genet. 2013. PMID: 23449626 Free PMC article.
-
Inactivation of Pink1 gene in vivo sensitizes dopamine-producing neurons to 1-methyl-4-phenyl-1,2,3,6-tetrahydropyridine (MPTP) and can be rescued by autosomal recessive Parkinson disease genes, Parkin or DJ-1.J Biol Chem. 2012 Jun 29;287(27):23162-70. doi: 10.1074/jbc.M112.346437. Epub 2012 Apr 17. J Biol Chem. 2012. PMID: 22511790 Free PMC article.
-
Pathway-specific dopaminergic deficits in a mouse model of Angelman syndrome.J Clin Invest. 2012 Dec;122(12):4544-54. doi: 10.1172/JCI61888. Epub 2012 Nov 12. J Clin Invest. 2012. PMID: 23143301 Free PMC article.
References
-
- Kitada T, Asakawa S, Hattori N, Matsumine H, Yamamura Y, Minoshima S, Yokochi M, Mizuno Y, Shimizu N. Nature. 1998;392:605–608. - PubMed
-
- Bonifati V, Rizzu P, van Baren MJ, Schaap O, Breedveld GJ, Krieger E, Dekker MC, Squitieri F, Ibanez P, Joosse M, et al. Science. 2003;299:256–259. - PubMed
-
- Valente EM, Abou-Sleiman PM, Caputo V, Muqit MM, Harvey K, Gispert S, Ali Z, Del Turco D, Bentivoglio AR, Healy DG, et al. Science. 2004;304:1158–1160. - PubMed
-
- Unoki M, Nakamura Y. Oncogene. 2001;20:4457–4465. - PubMed
-
- Rohe CF, Montagna P, Breedveld G, Cortelli P, Oostra BA, Bonifati V. Ann Neurol. 2004;56:427–431. - PubMed
Publication types
MeSH terms
Substances
LinkOut - more resources
Full Text Sources
Molecular Biology Databases
Research Materials