Selective redox regulation of cytokine receptor signaling by extracellular thioredoxin-1
- PMID: 17557078
- PMCID: PMC1914094
- DOI: 10.1038/sj.emboj.7601746
Selective redox regulation of cytokine receptor signaling by extracellular thioredoxin-1
Abstract
The thiol-disulfide oxidoreductase thioredoxin-1 (Trx1) is known to be secreted by leukocytes and to exhibit cytokine-like properties. Extracellular effects of Trx1 require a functional active site, suggesting a redox-based mechanism of action. However, specific cell surface proteins and pathways coupling extracellular Trx1 redox activity to cellular responses have not been identified so far. Using a mechanism-based kinetic trapping technique to identify disulfide exchange interactions on the intact surface of living lymphocytes, we found that Trx1 catalytically interacts with a single principal target protein. This target protein was identified as the tumor necrosis factor receptor superfamily member 8 (TNFRSF8/CD30). We demonstrate that the redox interaction is highly specific for both Trx1 and CD30 and that the redox state of CD30 determines its ability to engage the cognate ligand and transduce signals. Furthermore, we confirm that Trx1 affects CD30-dependent changes in lymphocyte effector function. Thus, we conclude that receptor-ligand signaling interactions can be selectively regulated by an extracellular redox catalyst.
Figures
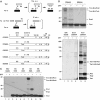
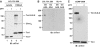
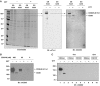
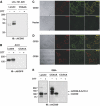
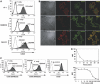
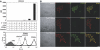
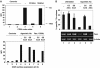
Similar articles
-
Regulation of interleukin-4 signaling by extracellular reduction of intramolecular disulfides.Biochem Biophys Res Commun. 2009 Dec 25;390(4):1272-7. doi: 10.1016/j.bbrc.2009.10.134. Epub 2009 Oct 28. Biochem Biophys Res Commun. 2009. PMID: 19878651
-
Identification of redox-active cell-surface proteins by mechanism-based kinetic trapping.Sci STKE. 2007 Dec 18;2007(417):pl8. doi: 10.1126/stke.4172007pl8. Sci STKE. 2007. PMID: 18089859
-
Thioredoxin fusions increase folding of single chain Fv antibodies in the cytoplasm of Escherichia coli: evidence that chaperone activity is the prime effect of thioredoxin.J Mol Biol. 2006 Mar 17;357(1):49-61. doi: 10.1016/j.jmb.2005.12.058. Epub 2006 Jan 6. J Mol Biol. 2006. PMID: 16427080
-
The extracellular microenvironment plays a key role in regulating the redox status of cell surface proteins in HIV-infected subjects.Arch Biochem Biophys. 2005 Feb 1;434(1):26-32. doi: 10.1016/j.abb.2004.11.015. Arch Biochem Biophys. 2005. PMID: 15629105 Review.
-
Introduction of the disulfide proteome: application of a technique for the analysis of plant storage proteins as well as allergens.J Proteome Res. 2008 Aug;7(8):3071-9. doi: 10.1021/pr8003453. Epub 2008 Jul 15. J Proteome Res. 2008. PMID: 18624400 Review.
Cited by
-
Peroxiredoxins wear many hats: Factors that fashion their peroxide sensing personalities.Redox Biol. 2021 Jun;42:101959. doi: 10.1016/j.redox.2021.101959. Epub 2021 Apr 20. Redox Biol. 2021. PMID: 33895094 Free PMC article. Review.
-
Mass Spectrometry in Advancement of Redox Precision Medicine.Adv Exp Med Biol. 2019;1140:327-358. doi: 10.1007/978-3-030-15950-4_19. Adv Exp Med Biol. 2019. PMID: 31347057 Free PMC article. Review.
-
Redox regulation of methionine aminopeptidase 2 activity.J Biol Chem. 2014 May 23;289(21):15035-43. doi: 10.1074/jbc.M114.554253. Epub 2014 Apr 3. J Biol Chem. 2014. PMID: 24700462 Free PMC article.
-
A role for 2-Cys peroxiredoxins in facilitating cytosolic protein thiol oxidation.Nat Chem Biol. 2018 Feb;14(2):148-155. doi: 10.1038/nchembio.2536. Epub 2017 Dec 18. Nat Chem Biol. 2018. PMID: 29251718 Free PMC article.
-
Arrest Functions of the MIF Ligand/Receptor Axes in Atherogenesis.Front Immunol. 2013 May 16;4:115. doi: 10.3389/fimmu.2013.00115. eCollection 2013. Front Immunol. 2013. PMID: 23720662 Free PMC article.
References
-
- Bertini R, Howard OM, Dong HF, Oppenheim JJ, Bizzarri C, Sergi R, Caselli G, Pagliei S, Romines B, Wilshire JA, Mengozzi M, Nakamura H, Yodoi J, Pekkari K, Gurunath R, Holmgren A, Herzenberg LA, Ghezzi P (1999) Thioredoxin, a redox enzyme released in infection and inflammation, is a unique chemoattractant for neutrophils, monocytes, and T cells. J Exp Med 189: 1783–1789 - PMC - PubMed
-
- Bodmer JL, Schneider P, Tschopp J (2002) The molecular architecture of the TNF superfamily. Trends Biochem Sci 27: 19–26 - PubMed
-
- Bowen MA, Olsen KJ, Cheng L, Avila D, Podack ER (1993) Functional effects of CD30 on a large granular lymphoma cell line, YT. Inhibition of cytotoxicity, regulation of CD28 and IL-2R, and induction of homotypic aggregation. J Immunol 151: 5896–5906 - PubMed
Publication types
MeSH terms
Substances
LinkOut - more resources
Full Text Sources
Other Literature Sources
Molecular Biology Databases
Research Materials
Miscellaneous