Noncovalent interaction between Ubc9 and SUMO promotes SUMO chain formation
- PMID: 17491593
- PMCID: PMC1888673
- DOI: 10.1038/sj.emboj.7601711
Noncovalent interaction between Ubc9 and SUMO promotes SUMO chain formation
Abstract
The ubiquitin-related modifier SUMO regulates a wide range of cellular processes by post-translational modification with one, or a chain of SUMO molecules. Sumoylation is achieved by the sequential action of several enzymes in which the E2, Ubc9, transfers SUMO from the E1 to the target mostly with the help of an E3 enzyme. In this process, Ubc9 not only forms a thioester bond with SUMO, but also interacts with SUMO noncovalently. Here, we show that this noncovalent interaction promotes the formation of short SUMO chains on targets such as Sp100 and HDAC4. We present a crystal structure of the noncovalent Ubc9-SUMO1 complex, showing that SUMO is located far from the E2 active site and resembles the noncovalent interaction site for ubiquitin on UbcH5c and Mms2. Structural comparison suggests a model for poly-sumoylation involving a mechanism analogous to Mms2-Ubc13-mediated ubiquitin chain formation.
Figures
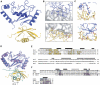
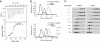
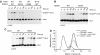
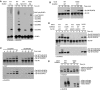
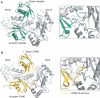
Similar articles
-
Depressing time: Waiting, melancholia, and the psychoanalytic practice of care.In: Kirtsoglou E, Simpson B, editors. The Time of Anthropology: Studies of Contemporary Chronopolitics. Abingdon: Routledge; 2020. Chapter 5. In: Kirtsoglou E, Simpson B, editors. The Time of Anthropology: Studies of Contemporary Chronopolitics. Abingdon: Routledge; 2020. Chapter 5. PMID: 36137063 Free Books & Documents. Review.
-
Comparison of Two Modern Survival Prediction Tools, SORG-MLA and METSSS, in Patients With Symptomatic Long-bone Metastases Who Underwent Local Treatment With Surgery Followed by Radiotherapy and With Radiotherapy Alone.Clin Orthop Relat Res. 2024 Dec 1;482(12):2193-2208. doi: 10.1097/CORR.0000000000003185. Epub 2024 Jul 23. Clin Orthop Relat Res. 2024. PMID: 39051924
-
Qualitative evidence synthesis informing our understanding of people's perceptions and experiences of targeted digital communication.Cochrane Database Syst Rev. 2019 Oct 23;10(10):ED000141. doi: 10.1002/14651858.ED000141. Cochrane Database Syst Rev. 2019. PMID: 31643081 Free PMC article.
-
Unlocking data: Decision-maker perspectives on cross-sectoral data sharing and linkage as part of a whole-systems approach to public health policy and practice.Public Health Res (Southampt). 2024 Nov 20:1-30. doi: 10.3310/KYTW2173. Online ahead of print. Public Health Res (Southampt). 2024. PMID: 39582242
-
Pharmacological treatments in panic disorder in adults: a network meta-analysis.Cochrane Database Syst Rev. 2023 Nov 28;11(11):CD012729. doi: 10.1002/14651858.CD012729.pub3. Cochrane Database Syst Rev. 2023. PMID: 38014714 Free PMC article. Review.
Cited by
-
A MUB E2 structure reveals E1 selectivity between cognate ubiquitin E2s in eukaryotes.Nat Commun. 2016 Aug 23;7:12580. doi: 10.1038/ncomms12580. Nat Commun. 2016. PMID: 27550514 Free PMC article.
-
Noncanonical E2 recruitment by the autophagy E1 revealed by Atg7-Atg3 and Atg7-Atg10 structures.Nat Struct Mol Biol. 2012 Dec;19(12):1242-9. doi: 10.1038/nsmb.2415. Epub 2012 Nov 11. Nat Struct Mol Biol. 2012. PMID: 23142976 Free PMC article.
-
Biochemistry: Rear view of an enzyme.Nature. 2013 May 30;497(7451):576-7. doi: 10.1038/nature12249. Epub 2013 May 22. Nature. 2013. PMID: 23698365 No abstract available.
-
Mechanistic insights into active site-associated polyubiquitination by the ubiquitin-conjugating enzyme Ube2g2.Proc Natl Acad Sci U S A. 2009 Mar 10;106(10):3722-7. doi: 10.1073/pnas.0808564106. Epub 2009 Feb 17. Proc Natl Acad Sci U S A. 2009. PMID: 19223579 Free PMC article.
-
Alterations of ubiquitylation and sumoylation in conventional renal cell carcinomas after the Chernobyl accident: a comparison with Spanish cases.Virchows Arch. 2011 Sep;459(3):307-13. doi: 10.1007/s00428-011-1124-8. Epub 2011 Jul 23. Virchows Arch. 2011. PMID: 21786080
References
-
- Bencsath KP, Podgorski MS, Pagala VR, Slaughter CA, Schulman BA (2002) Identification of a multifunctional binding site on Ubc9p required for Smt3p conjugation. J Biol Chem 277: 47938–47945 - PubMed
-
- Bernier-Villamor V, Sampson DA, Matunis MJ, Lima CD (2002) Structural basis for E2-mediated SUMO conjugation revealed by a complex between ubiquitin-conjugating enzyme Ubc9 and RanGAP1. Cell 108: 1908 - PubMed
-
- Brzovic PS, Lissounov A, Christensen DE, Hoyt DW, Klevit RE (2006) A UbcH5/ubiquitin noncovalent complex is required for processive BRCA1-directed ubiquitination. Mol Cell 21: 873–880 - PubMed
-
- Bylebyl GR, Belichenko I, Johnson ES (2003) The SUMO isopeptidase Ulp2 prevents accumulation of SUMO chains in yeast. J Biol Chem 278: 44113–44120 - PubMed
Publication types
MeSH terms
Substances
LinkOut - more resources
Full Text Sources
Other Literature Sources
Molecular Biology Databases
Miscellaneous