Shear-induced unfolding triggers adhesion of von Willebrand factor fibers
- PMID: 17470810
- PMCID: PMC1876544
- DOI: 10.1073/pnas.0608422104
Shear-induced unfolding triggers adhesion of von Willebrand factor fibers
Abstract
von Willebrand factor (VWF), a protein present in our circulatory system, is necessary to stop bleeding under high shear-stress conditions as found in small blood vessels. The results presented here help unravel how an increase in hydrodynamic shear stress activates VWF's adhesion potential, leading to the counterintuitive phenomena of enhanced adsorption rate under strong shear conditions. Using a microfluidic device, we were able to mimic a wide range of bloodflow conditions and directly visualize the conformational dynamics of this protein under shear flow. In particular, we find that VWF displays a reversible globule-stretch transition at a critical shear rate gamma(crit) in the absence of any adsorbing surface. Computer simulations reproduce this sharp transition and identify the large size of VWF's repeating units as one of the keys for this unique hydrodynamic activation. In the presence of an adsorbing collagen substrate, we find a large increase in the protein adsorption at the same critical shear rate, suggesting that the globule unfolding in bulk triggers the surface adsorption in the case of a collagen substrate, which provides a sufficient density of binding sites. Monitoring the adsorption process of multiple VWF fibers, we were able to follow the formation of an immobilized network that constitutes a "sticky" grid necessary for blood platelet adhesion under high shear flow. Because areas of high shear stress coincide with a higher chance for vessel wall damage by mechanical forces, we identified the shear-induced increase in the binding probability of VWF as an effective self-regulating repair mechanism of our microvascular system.
Conflict of interest statement
The authors declare no conflict of interest.
Figures
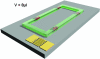
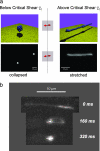
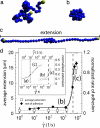
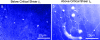
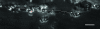
Similar articles
-
The effect of shear stress on protein conformation: Physical forces operating on biochemical systems: The case of von Willebrand factor.Biophys Chem. 2010 Dec;153(1):1-8. doi: 10.1016/j.bpc.2010.07.002. Epub 2010 Aug 11. Biophys Chem. 2010. PMID: 20797815 Review.
-
Are integrin alpha(2)beta(1), glycoprotein Ib and vWf levels correlated with their contributions to platelet adhesion on collagen under high-shear flow?Platelets. 2010;21(2):101-11. doi: 10.3109/09537100903497549. Platelets. 2010. PMID: 20063990
-
The interplay between adsorption and aggregation of von Willebrand factor chains in shear flows.Biophys J. 2023 Oct 3;122(19):3831-3842. doi: 10.1016/j.bpj.2023.07.028. Epub 2023 Aug 3. Biophys J. 2023. PMID: 37537863 Free PMC article.
-
Shear-stress-induced conformational changes of von Willebrand factor in a water-glycerol mixture observed with single molecule microscopy.J Phys Chem B. 2014 May 29;118(21):5660-9. doi: 10.1021/jp5022664. Epub 2014 May 15. J Phys Chem B. 2014. PMID: 24754487
-
Platelet-vessel wall interactions in thrombosis and restenosis role of von Willebrand factor.Verh K Acad Geneeskd Belg. 1997;59(3):161-83. Verh K Acad Geneeskd Belg. 1997. PMID: 9490916 Review.
Cited by
-
Modelling of platelet-fibrin clot formation in flow with a DPD-PDE method.J Math Biol. 2016 Feb;72(3):649-81. doi: 10.1007/s00285-015-0891-2. Epub 2015 May 24. J Math Biol. 2016. PMID: 26001742
-
Mechanical activation of a multimeric adhesive protein through domain conformational change.Phys Rev Lett. 2013 Mar 8;110(10):108102. doi: 10.1103/PhysRevLett.110.108102. Epub 2013 Mar 5. Phys Rev Lett. 2013. PMID: 23521301 Free PMC article.
-
Direct observation of von Willebrand factor elongation and fiber formation on collagen during acute whole blood exposure to pathological flow.Arterioscler Thromb Vasc Biol. 2013 Jan;33(1):105-13. doi: 10.1161/ATVBAHA.112.300522. Epub 2012 Oct 25. Arterioscler Thromb Vasc Biol. 2013. PMID: 23104847 Free PMC article.
-
Predicting pathological von Willebrand factor unraveling in elongational flow.Biophys J. 2021 May 18;120(10):1903-1915. doi: 10.1016/j.bpj.2021.03.008. Epub 2021 Mar 16. Biophys J. 2021. PMID: 33737157 Free PMC article.
-
Regulated unfolding of proteins in signaling.FEBS Lett. 2013 Apr 17;587(8):1081-8. doi: 10.1016/j.febslet.2013.02.024. Epub 2013 Feb 20. FEBS Lett. 2013. PMID: 23454209 Free PMC article. Review.
References
-
- Trusky GA, Yuan F, Katz DF. Transport Phemomena in Biological Systems. Upper Saddle River, New Jersey: Pearson Education; 2004.
-
- Lorz B, Simson R, Nardi J, Sackmann E. Europhys Lett. 2000;51:468–474.
-
- Goldmann AJ, Cox RG, Brenner H. Chem Eng Sci. 1967;22:653–660.
-
- Seifert U. Phys Rev Lett. 1999;83:876–879.
Publication types
MeSH terms
Substances
LinkOut - more resources
Full Text Sources
Other Literature Sources
Miscellaneous