Structural evidence for regulation and specificity of flaviviral proteases and evolution of the Flaviviridae fold
- PMID: 17400917
- PMCID: PMC2206648
- DOI: 10.1110/ps.072753207
Structural evidence for regulation and specificity of flaviviral proteases and evolution of the Flaviviridae fold
Abstract
Pathogenic members of the flavivirus family, including West Nile Virus (WNV) and Dengue Virus (DV), are growing global threats for which there are no specific treatments. The two-component flaviviral enzyme NS2B-NS3 cleaves the viral polyprotein precursor within the host cell, a process that is required for viral replication. Here, we report the crystal structure of WNV NS2B-NS3pro both in a substrate-free form and in complex with the trypsin inhibitor aprotinin/BPTI. We show that aprotinin binds in a substrate-mimetic fashion in which the productive conformation of the protease is fully formed, providing evidence for an "induced fit" mechanism of catalysis and allowing us to rationalize the distinct substrate specificities of WNV and DV proteases. We also show that the NS2B cofactor of WNV can adopt two very distinct conformations and that this is likely to be a general feature of flaviviral proteases, providing further opportunities for regulation. Finally, by comparing the flaviviral proteases with the more distantly related Hepatitis C virus, we provide insights into the evolution of the Flaviviridae fold. Our work should expedite the design of protease inhibitors to treat a range of flaviviral infections.
Figures
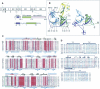
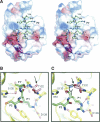
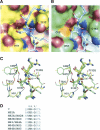
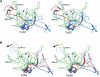
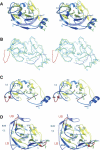
Similar articles
-
Homology modeling and molecular dynamics simulations of Dengue virus NS2B/NS3 protease: insight into molecular interaction.J Mol Recognit. 2010 May-Jun;23(3):283-300. doi: 10.1002/jmr.977. J Mol Recognit. 2010. PMID: 19693793
-
Structural basis for the activation of flaviviral NS3 proteases from dengue and West Nile virus.Nat Struct Mol Biol. 2006 Apr;13(4):372-3. doi: 10.1038/nsmb1073. Epub 2006 Mar 12. Nat Struct Mol Biol. 2006. PMID: 16532006
-
Crystal structure of Dengue virus NS3 protease in complex with a Bowman-Birk inhibitor: implications for flaviviral polyprotein processing and drug design.J Mol Biol. 2000 Aug 25;301(4):759-67. doi: 10.1006/jmbi.2000.3924. J Mol Biol. 2000. Retraction in: J Mol Biol. 2010 Apr 9;397(4):1119. doi: 10.1016/j.jmb.2010.01.069 PMID: 10966782 Retracted.
-
The Transactions of NS3 and NS5 in Flaviviral RNA Replication.Adv Exp Med Biol. 2018;1062:147-163. doi: 10.1007/978-981-10-8727-1_11. Adv Exp Med Biol. 2018. PMID: 29845531 Review.
-
Architects of assembly: roles of Flaviviridae non-structural proteins in virion morphogenesis.Nat Rev Microbiol. 2008 Sep;6(9):699-708. doi: 10.1038/nrmicro1928. Nat Rev Microbiol. 2008. PMID: 18587411 Free PMC article. Review.
Cited by
-
Antiviral potential of 4-hydroxypanduratin A, secondary metabolite of Fingerroot, Boesenbergia pandurata (Schult.), towards Japanese Encephalitis virus NS2B/NS3 protease.Bioinformation. 2013;9(1):54-60. doi: 10.6026/97320630009054. Epub 2013 Jan 9. Bioinformation. 2013. PMID: 23390345 Free PMC article.
-
Switching the substrate specificity of the two-component NS2B-NS3 flavivirus proteinase by structure-based mutagenesis.J Virol. 2007 May;81(9):4501-9. doi: 10.1128/JVI.02719-06. Epub 2007 Feb 14. J Virol. 2007. PMID: 17301157 Free PMC article.
-
Design, synthesis, and in vitro evaluation of potential West Nile virus protease inhibitors based on the 1-oxo-1,2,3,4-tetrahydroisoquinoline and 1-oxo-1,2-dihydroisoquinoline scaffolds.J Comb Chem. 2010 Nov 8;12(6):836-43. doi: 10.1021/cc100091h. Epub 2010 Sep 30. J Comb Chem. 2010. PMID: 20882963 Free PMC article.
-
Virtual ligand screening of the National Cancer Institute (NCI) compound library leads to the allosteric inhibitory scaffolds of the West Nile Virus NS3 proteinase.Assay Drug Dev Technol. 2011 Feb;9(1):69-78. doi: 10.1089/adt.2010.0309. Epub 2010 Nov 4. Assay Drug Dev Technol. 2011. PMID: 21050032 Free PMC article.
-
Potential Role of Flavivirus NS2B-NS3 Proteases in Viral Pathogenesis and Anti-flavivirus Drug Discovery Employing Animal Cells and Models: A Review.Viruses. 2021 Dec 28;14(1):44. doi: 10.3390/v14010044. Viruses. 2021. PMID: 35062249 Free PMC article. Review.
References
-
- Blaney J.E., Manipon, G.G., Murphy, B.R., and Whitehead, S.S. 2003. Temperature sensitive mutations in the genes encoding the NS1, NS2A, NS3, and NS5 nonstructural proteins of dengue virus type 4 restrict replication in the brains of mice. Arch. Virol. 148: 999–1006. - PubMed
-
- Bricogne G., Vonrhein, C., Flensburg, C., Schiltz, M., and Paciorek, W. 2003. Generation, representation and flow of phase information in structure determination: Recent developments in and around SHARP 2.0. Acta Crystallogr. D Biol. Crystallogr. 59: 2023–2030. - PubMed
-
- Brünger A.T., Adams, P.D., Clore, G.M., DeLano, W.L., Gros, P., Grosse-Kunstleve, R.W., Jiang, J.S., Kuszewski, J., Nilges, M., Pannu, N.S., et al. 1998. Crystallography & NMR system: A new software suite for macromolecular structure determination. Acta Crystallogr. D Biol. Crystallogr. 54: 905–921. - PubMed
-
- Burke D.S. and Monath, T.P. 2001. Flaviviruses. In Fields Virology (eds. D.M. Knipe and P.M. Howley), pp. 1043–1125. Lippincott Williams & Wikins, Philadelphia.
-
- Chambers T.J., Weir, R.C., Grakoui, A., McCourt, D.W., Bazan, J.F., Fletterick, R.J., and Rice, C.M. 1990. Evidence that the N-terminal domain of nonstructural protein NS3 from yellow fever virus is a serine protease responsible for site-specific cleavages in the viral polyprotein. Proc. Natl. Acad. Sci. 87: 8898–8902. - PMC - PubMed
Publication types
MeSH terms
Substances
Grants and funding
LinkOut - more resources
Full Text Sources
Other Literature Sources