Slack and Slick K(Na) channels regulate the accuracy of timing of auditory neurons
- PMID: 17344399
- PMCID: PMC6672517
- DOI: 10.1523/JNEUROSCI.5308-06.2007
Slack and Slick K(Na) channels regulate the accuracy of timing of auditory neurons
Abstract
The Slack (sequence like a calcium-activated K channel) and Slick (sequence like an intermediate conductance K channel) genes, which encode sodium-activated K+ (K(Na)) channels, are expressed at high levels in neurons of the medial nucleus of the trapezoid body (MNTB) in the auditory brainstem. These neurons lock their action potentials to incoming stimuli with a high degree of temporal precision. Channels with unitary properties similar to those of Slack and/or Slick channels, which are gated by [Na+]i and [Cl-]i and by changes in cytoplasmic ATP levels, are present in MNTB neurons. Manipulations of the level of K(Na) current in MNTB neurons, either by increasing levels of internal Na+ or by exposure to a pharmacological activator of Slack channels, significantly enhance the accuracy of timing of action potentials at high frequencies of stimulation. These findings suggest that such fidelity of timing at high frequencies may be attributed in part to high-conductance K(Na) channels.
Figures
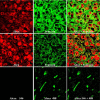
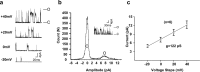
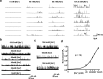
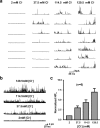
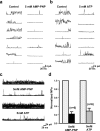
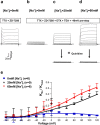
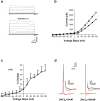
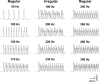
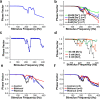
Similar articles
-
Localization of the Na+-activated K+ channel Slick in the rat central nervous system.J Comp Neurol. 2005 Mar 28;484(1):80-92. doi: 10.1002/cne.20462. J Comp Neurol. 2005. PMID: 15717307
-
Slack and Slick KNa channels are required for the depolarizing afterpotential of acutely isolated, medium diameter rat dorsal root ganglion neurons.Acta Pharmacol Sin. 2008 Aug;29(8):899-905. doi: 10.1111/j.1745-7254.2008.00842.x. Acta Pharmacol Sin. 2008. PMID: 18664322
-
Regulation of the timing of MNTB neurons by short-term and long-term modulation of potassium channels.Hear Res. 2005 Aug;206(1-2):133-45. doi: 10.1016/j.heares.2004.11.023. Hear Res. 2005. PMID: 16081004 Review.
-
Systematic variation of potassium current amplitudes across the tonotopic axis of the rat medial nucleus of the trapezoid body.Hear Res. 2005 Aug;206(1-2):116-32. doi: 10.1016/j.heares.2004.12.012. Hear Res. 2005. PMID: 16081003
-
For K+ channels, Na+ is the new Ca2+.Trends Neurosci. 2005 Aug;28(8):422-8. doi: 10.1016/j.tins.2005.06.003. Trends Neurosci. 2005. PMID: 15979166 Review.
Cited by
-
Cardiac metabolic effects of KNa1.2 channel deletion and evidence for its mitochondrial localization.FASEB J. 2018 Jun 4;32(11):fj201800139R. doi: 10.1096/fj.201800139R. Online ahead of print. FASEB J. 2018. PMID: 29863912 Free PMC article.
-
Cell volume changes regulate slick (Slo2.1), but not slack (Slo2.2) K+ channels.PLoS One. 2014 Oct 27;9(10):e110833. doi: 10.1371/journal.pone.0110833. eCollection 2014. PLoS One. 2014. PMID: 25347289 Free PMC article.
-
Cryo-electron microscopy structure of the Slo2.2 Na(+)-activated K(+) channel.Nature. 2015 Nov 12;527(7577):198-203. doi: 10.1038/nature14958. Epub 2015 Oct 5. Nature. 2015. PMID: 26436452 Free PMC article.
-
Epilepsy-Related Slack Channel Mutants Lead to Channel Over-Activity by Two Different Mechanisms.Cell Rep. 2016 Jan 5;14(1):129-139. doi: 10.1016/j.celrep.2015.12.019. Epub 2015 Dec 24. Cell Rep. 2016. PMID: 26725113 Free PMC article.
-
TMEM16C facilitates Na(+)-activated K+ currents in rat sensory neurons and regulates pain processing.Nat Neurosci. 2013 Sep;16(9):1284-90. doi: 10.1038/nn.3468. Epub 2013 Jul 21. Nat Neurosci. 2013. PMID: 23872594 Free PMC article.
References
Publication types
MeSH terms
Substances
Grants and funding
LinkOut - more resources
Full Text Sources
Molecular Biology Databases